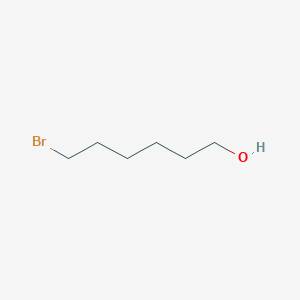
6-Bromo-1-hexanol
Overview
Description
6-Bromo-1-hexanol (CAS: 4286-55-9) is a bifunctional organic compound with the molecular formula C₆H₁₃BrO and a molecular weight of 181.07 g/mol . It features a hydroxyl (-OH) group at the terminal carbon and a bromine atom at the sixth carbon, enabling dual reactivity in nucleophilic substitutions and esterification reactions. This compound is widely used in organic synthesis, including the preparation of ionic liquids (e.g., 1-(6-hydroxyhexyl)-3-methylimidazolium bromide) , polymers , and pharmaceutical intermediates . Its density is 1.384 g/mL at 25°C, with a boiling point of 105–106°C at 5 mmHg and a refractive index of 1.482 .
Preparation Methods
Halogenation of 1,6-Hexanediol with Hydrobromic Acid
The most widely documented method for synthesizing 6-bromo-1-hexanol involves the halogenation of 1,6-hexanediol using hydrobromic acid (HBr). This approach, detailed in the patent CN102633619A , offers high selectivity and scalability.
Reaction Mechanism
The reaction proceeds via nucleophilic substitution (SN2), where one hydroxyl group of 1,6-hexanediol is replaced by a bromine atom. The process occurs in two stages:
-
Protonation : Sulfuric acid protonates the hydroxyl group, converting it into a better-leaving water molecule.
-
Substitution : Bromide ion (from HBr) attacks the adjacent carbon, displacing water to form this compound.
The chemical equation is:
2\text{SO}4, \Delta} \text{this compound} + \text{H}_2\text{O}
Optimization of Reaction Conditions
Key parameters influencing yield and purity include:
Example Synthesis (Patent CN102633619A ) :
-
Reactants : 20 g 1,6-hexanediol (0.169 mol), 21 mL 47% HBr (0.182 mol).
-
Conditions : Toluene solvent, 80–85°C, 8 hours.
-
Workup : Distillation under reduced pressure (70 kPa) yields this compound (155–165°C fraction).
Purification Techniques
-
Distillation : Removes unreacted diol and solvent.
-
Washing : Neutralizes residual acid with aqueous NaOH.
-
Drying : Anhydrous MgSO₄ ensures moisture-free product.
Alternative Synthetic Routes
While the halogenation of 1,6-hexanediol dominates industrial production, alternative methods have been explored:
Hydrobromination of 1-Hexanol (Theoretical Approach)
A proposed method involves reacting 1-hexanol with HBr under acidic conditions. However, this route predominantly yields 1-bromohexane due to the primary alcohol’s high reactivity, making it impractical for this compound synthesis .
Enzymatic Bromination
Emerging research explores lipase-catalyzed bromination in non-aqueous media, though yields remain suboptimal (<50%) compared to traditional methods.
Industrial Production Considerations
Large-scale synthesis prioritizes cost-efficiency and safety:
-
Continuous Flow Reactors : Enhance heat transfer and reduce reaction time.
-
Waste Management : HBr recovery systems minimize environmental impact.
-
Quality Control : GC-MS and NMR validate purity (>98%).
Comparative Analysis of Methods
Method | Yield | Scalability | Cost | Environmental Impact |
---|---|---|---|---|
1,6-Hexanediol + HBr | 85–90% | High | Low | Moderate (HBr waste) |
Enzymatic | 40–50% | Low | High | Low |
Applications of this compound
Chemical Reactions Analysis
6-Bromo-1-hexanol undergoes several types of chemical reactions, including:
Nucleophilic Substitution: The bromine atom in this compound is a good leaving group, making it susceptible to nucleophilic substitution reactions.
Oxidation: The hydroxyl group can be oxidized to form the corresponding aldehyde or carboxylic acid under appropriate conditions.
Reduction: The compound can be reduced to form 1-hexanol by removing the bromine atom.
Esterification: The hydroxyl group can react with carboxylic acids or their derivatives to form esters.
Common reagents used in these reactions include sodium azide, phenol, carbon dioxide, and various oxidizing and reducing agents . Major products formed from these reactions include 6-azido-1-hexanol, 6-phenoxy-1-hexanol, and 6-diethylamino-1-hexanol .
Scientific Research Applications
Proteomics Research
6-Bromo-1-hexanol is utilized in proteomics for the preparation of nitric oxide-donating analogues of Sulindac, a non-steroidal anti-inflammatory drug (NSAID). This application is crucial for studying potential anti-cancer properties related to colon cancer prevention .
Synthesis of Oligonucleotides
The compound acts as a cross-linker in the synthesis of cyclic, branched, and bicyclic oligonucleotides through click chemistry. This method enhances the stability and functionality of nucleic acid constructs, which are essential in genetic research and therapeutic development .
Synthesis of Bisphosphonates
Recent studies have explored the use of this compound in synthesizing bisphosphonates, compounds widely used for their medicinal properties, particularly in treating bone diseases. The synthesis involves treating brominated hexanoic acid with phosphonic acid derivatives, demonstrating the compound's relevance in pharmaceutical chemistry .
Case Study 1: Nitric Oxide Donating Analogs
A study highlighted the role of this compound in creating nitric oxide-donating analogs of Sulindac. These analogs were evaluated for their ability to induce apoptosis in cancer cells, showcasing potential therapeutic benefits beyond traditional NSAIDs .
Case Study 2: Oligonucleotide Synthesis
In a research project focused on developing novel oligonucleotide structures, researchers employed this compound as a cross-linker. The resulting cyclic oligonucleotides exhibited enhanced binding affinity and stability compared to linear counterparts, indicating significant advancements in nucleic acid technology .
Mechanism of Action
The mechanism of action of 6-Bromo-1-hexanol is primarily attributed to the reactivity of the bromine atom within the molecule. The bromine atom serves as a nucleophile, initiating substrate attack and generating a bromonium ion. This bromonium ion can undergo diverse reactions depending on the reaction conditions . The hydroxyl group also enables further derivatization or replacement with other reactive functional groups .
Comparison with Similar Compounds
This section compares 6-bromo-1-hexanol with structurally analogous bromoalcohols (e.g., 8-bromo-1-octanol, 10-bromo-1-decanol) and haloalcohols (e.g., 6-chloro-1-hexanol) across physical properties, reactivity, synthetic performance, and toxicity.
Physical and Chemical Properties
Compound | Molecular Formula | Molecular Weight (g/mol) | Boiling Point (°C/mmHg) | Density (g/mL) | Refractive Index |
---|---|---|---|---|---|
This compound | C₆H₁₃BrO | 181.07 | 105–106 (5 mmHg) | 1.384 | 1.482 |
8-Bromo-1-octanol | C₈H₁₇BrO | 209.12 | ~130–135 (5 mmHg)* | ~1.25* | ~1.48* |
10-Bromo-1-decanol | C₁₀H₂₁BrO | 237.17 | ~160–165 (5 mmHg)* | ~1.20* | ~1.48* |
6-Chloro-1-hexanol | C₆H₁₃ClO | 136.62 | ~85–90 (5 mmHg)* | ~1.12* | ~1.45* |
*Estimated based on homologous series trends.
Key Observations :
- Chain Length Effects: Longer bromoalcohols (e.g., 8-bromo-1-octanol, 10-bromo-1-decanol) exhibit higher molecular weights and boiling points due to increased van der Waals interactions .
- Halogen Substitution: Bromine’s higher atomic weight and polarizability compared to chlorine result in greater density and refractive index for this compound versus 6-chloro-1-hexanol .
Nucleophilic Substitution
- This compound’s bromine atom acts as a superior leaving group compared to chlorine in 6-chloro-1-hexanol, enabling faster alkylation and quaternization reactions (e.g., synthesis of imidazolium ionic liquids) .
- In glycosylation reactions, this compound yielded 24–40% bromoalkyl glycosides using microreactor systems, outperforming 8-bromo-1-octanol (27%) and 10-bromo-1-decanol (18%) in traditional setups .
Impurity Profiles
- This compound often contains dibromide impurities (e.g., 1,6-dibromohexane) due to incomplete monobromination during synthesis. These impurities complicate purification in sensitive applications, such as lipid nanoparticle production for vaccines .
- Longer bromoalcohols (8-bromo-1-octanol, 10-bromo-1-decanol) exhibit similar impurity challenges but are less frequently reported in pharmaceutical contexts .
Toxicity Comparison
Compound | log(IGC₅₀⁻¹)* | Toxicity Relative to this compound |
---|---|---|
This compound | 0.01 | Baseline |
8-Bromo-1-octanol | 1.04 | Less toxic |
6-Chloro-1-hexanol | -0.27 | More toxic |
2-Bromoethanol | -0.85 | More toxic |
*Higher log(IGC₅₀⁻¹) values correlate with lower toxicity. Data sourced from Tetrahymena pyriformis assays .
Key Observations :
- Chain Length: Toxicity decreases with longer carbon chains (e.g., 8-bromo-1-octanol > this compound) due to reduced bioavailability .
- Halogen Type: Chloroalcohols (e.g., 6-chloro-1-hexanol) are more toxic than bromoanalogs, likely due to stronger electrophilicity .
Industrial and Research Performance
Key Observations :
- Yield Improvements: Microreactor technology significantly enhances yields for longer bromoalcohols, making 8-bromo-1-octanol and 10-bromo-1-decanol more viable for industrial glycoside production .
- Catalyst Optimization: this compound synthesis benefits from iodine catalysts, which reduce reaction time and improve atom economy .
Biological Activity
6-Bromo-1-hexanol, a small molecule with the chemical formula CHBrO, has garnered attention in various fields, including medicinal chemistry and biochemistry, due to its unique structural properties and potential biological activities. This article aims to provide a comprehensive overview of its biological activity, including antimicrobial properties, cytotoxic effects, and potential mechanisms of action.
- Chemical Name: 6-bromohexan-1-ol
- CAS Number: 4286-55-9
- Molecular Weight: 181.07 g/mol
- Boiling Point: 105–106 °C (5 mmHg)
- Density: 1.384 g/mL at 25 °C
Biological Activity Overview
The biological activity of this compound has been studied in various contexts, particularly its antimicrobial and cytotoxic effects. Below are key findings from recent research.
Antimicrobial Activity
Research indicates that this compound exhibits significant antimicrobial properties. A study evaluated its efficacy against several bacterial strains, revealing:
Microorganism | Minimum Inhibitory Concentration (MIC) |
---|---|
Escherichia coli | 13 mg/mL |
Bacillus subtilis | 18 mg/mL |
Staphylococcus aureus | Notable inhibition at higher concentrations |
These results suggest that this compound could be effective in treating infections caused by these pathogens, although specific clinical applications remain to be established .
Cytotoxic Effects
In addition to its antimicrobial properties, this compound has shown cytotoxic effects on cancer cell lines. The compound was tested against various cancer types, including colorectal adenocarcinoma cell lines:
Cell Line | IC Value (µg/mL) |
---|---|
Caco-2 | 714.6 |
DLD-1 | 254.3 |
These findings indicate that the compound may induce apoptosis in cancer cells, suggesting a potential role in cancer therapy .
The mechanisms underlying the biological activity of this compound are not fully elucidated but may involve several pathways:
- Membrane Disruption: The compound may disrupt microbial membranes, leading to cell lysis.
- Reactive Oxygen Species (ROS) Generation: It could induce oxidative stress in cancer cells, contributing to cytotoxicity.
- Enzyme Inhibition: Potential inhibition of specific enzymes involved in cell proliferation or survival pathways.
Case Studies
Several studies have explored the biological effects of halogenated alcohols similar to this compound:
- A comparative study on halogenated alcohols demonstrated that compounds with bromine substituents often exhibited enhanced antimicrobial activity compared to their chlorine counterparts.
Q & A
Basic Research Questions
Q. What are the common synthetic routes for 6-Bromo-1-hexanol, and how can purity be optimized?
this compound is typically synthesized via nucleophilic substitution reactions. For example, bromination of 1,6-hexanediol using hydrobromic acid (HBr) under controlled conditions can yield the compound. Purity optimization involves post-synthesis purification via column chromatography (e.g., using RP silica gel with ethanol/water eluent) to remove dibromide impurities, which are common in commercial batches . Solvent selection (e.g., DMF or dichloromethane) and reaction temperature control are critical to minimize side reactions .
Q. What spectroscopic methods are used to characterize this compound?
Structural elucidation relies on:
- Mass Spectrometry (MS): The molecular ion peak at m/z 181 (M⁺) and fragmentation patterns (e.g., loss of -OH [m/z 164] and subsequent Br elimination [m/z 83]) confirm the bromoalcohol structure .
- ¹H NMR: Peaks at δ 1.4–1.6 ppm (methylene protons adjacent to Br) and δ 3.6 ppm (hydroxyl-bearing terminal -CH₂) are diagnostic .
- TLC: Used to monitor reaction progress and detect impurities (e.g., dibromides) .
Q. How should this compound be handled to ensure stability during experiments?
The compound is moisture-sensitive and prone to oxidation. Store under inert gas (N₂/Ar) at ambient temperatures, and avoid prolonged exposure to light. Use anhydrous solvents (e.g., DMF) for reactions to prevent hydrolysis. Safety protocols include wearing nitrile gloves and working in a fume hood due to its irritant properties .
Advanced Research Questions
Q. How do impurities in commercial this compound affect reaction outcomes, and how can they be mitigated?
Commercial samples often contain dibromide impurities (e.g., 1,6-dibromohexane) due to incomplete synthesis. These can compete in reactions (e.g., alkylation), leading to byproducts. Mitigation strategies include:
- Pre-purification: Column chromatography with RP silica gel .
- In-situ monitoring: TLC or GC-MS to track impurity levels during synthesis .
- Alternative synthesis: Microreactor systems (e.g., borosilicate reactors with Teflon tubing) improve reaction homogeneity and reduce impurity formation .
Q. What catalytic systems enhance the efficiency of this compound in glycosylation or esterification reactions?
- Glycosylation: Trimethylsilyl triflate (TMSOTf) outperforms H₂SO₄ or trifluoroacetic acid, yielding higher product purity (85–90% vs. 60–70%) by minimizing acid-catalyzed side reactions .
- Esterification: Use of DMAP (4-dimethylaminopyridine) as a catalyst in anhydrous dichloromethane improves acylation efficiency .
Q. How can continuous-flow microreactors improve the scalability of this compound synthesis?
Microreactors (e.g., borosilicate LTF V systems) enable precise control of residence time and temperature, critical for bromination reactions. Benefits include:
- Reduced side products: Enhanced mixing minimizes localized overheating, suppressing dibromide formation .
- Scalability: Modular setups allow parallelization for gram-to-kilogram synthesis without compromising yield .
Q. What mechanistic insights explain the stability of this compound under acidic vs. basic conditions?
Under acidic conditions, the hydroxyl group is protonated, reducing nucleophilicity and stabilizing the compound. In contrast, basic conditions deprotonate the -OH group, increasing susceptibility to elimination (forming 1-hexene) or substitution. Kinetic studies using deuterated solvents (e.g., D₂O) can track proton transfer pathways .
Q. How do isotopic labeling studies aid in tracing this compound’s metabolic or synthetic pathways?
¹³C or ²H labeling at the bromine-adjacent carbon enables tracking via NMR or MS. For example:
- Biodegradation studies: ¹³C-labeled this compound can monitor microbial degradation products in environmental samples.
- Reaction mechanisms: Deuterated analogs (e.g., this compound-d₂) reveal hydrogen-bonding effects in catalytic cycles .
Q. Data Contradiction Analysis
Q. Why do NMR and MS data sometimes conflict when characterizing this compound batches?
Discrepancies often arise from:
- Impurities: Dibromides or oxidation products (e.g., hexanal) can skew integration ratios in ¹H NMR .
- Solvent artifacts: Residual solvents (e.g., DMF) in MS may produce adduct peaks (e.g., [M+Na]⁺) .
- Deuterium exchange: In D₂O, the hydroxyl proton signal disappears, altering NMR interpretations .
Q. How can researchers resolve conflicting reactivity reports of this compound in literature?
Contradictory reactivity (e.g., variable yields in alkylation) may stem from:
- Batch variability: Commercial suppliers may have inconsistent impurity profiles .
- Reaction conditions: Moisture levels, solvent purity, or catalyst aging (e.g., TMSOTf hydrolysis) significantly impact outcomes .
- Validation: Reproduce experiments with rigorously purified samples and standardized protocols .
Q. Methodological Recommendations
Properties
IUPAC Name |
6-bromohexan-1-ol | |
---|---|---|
Source | PubChem | |
URL | https://pubchem.ncbi.nlm.nih.gov | |
Description | Data deposited in or computed by PubChem | |
InChI |
InChI=1S/C6H13BrO/c7-5-3-1-2-4-6-8/h8H,1-6H2 | |
Source | PubChem | |
URL | https://pubchem.ncbi.nlm.nih.gov | |
Description | Data deposited in or computed by PubChem | |
InChI Key |
FCMCSZXRVWDVAW-UHFFFAOYSA-N | |
Source | PubChem | |
URL | https://pubchem.ncbi.nlm.nih.gov | |
Description | Data deposited in or computed by PubChem | |
Canonical SMILES |
C(CCCBr)CCO | |
Source | PubChem | |
URL | https://pubchem.ncbi.nlm.nih.gov | |
Description | Data deposited in or computed by PubChem | |
Molecular Formula |
C6H13BrO | |
Source | PubChem | |
URL | https://pubchem.ncbi.nlm.nih.gov | |
Description | Data deposited in or computed by PubChem | |
DSSTOX Substance ID |
DTXSID2063397 | |
Record name | 1-Hexanol, 6-bromo- | |
Source | EPA DSSTox | |
URL | https://comptox.epa.gov/dashboard/DTXSID2063397 | |
Description | DSSTox provides a high quality public chemistry resource for supporting improved predictive toxicology. | |
Molecular Weight |
181.07 g/mol | |
Source | PubChem | |
URL | https://pubchem.ncbi.nlm.nih.gov | |
Description | Data deposited in or computed by PubChem | |
Physical Description |
Clear colorless to slightly yellow liquid; [Acros Organics MSDS] | |
Record name | 6-Bromo-1-hexanol | |
Source | Haz-Map, Information on Hazardous Chemicals and Occupational Diseases | |
URL | https://haz-map.com/Agents/19825 | |
Description | Haz-Map® is an occupational health database designed for health and safety professionals and for consumers seeking information about the adverse effects of workplace exposures to chemical and biological agents. | |
Explanation | Copyright (c) 2022 Haz-Map(R). All rights reserved. Unless otherwise indicated, all materials from Haz-Map are copyrighted by Haz-Map(R). No part of these materials, either text or image may be used for any purpose other than for personal use. Therefore, reproduction, modification, storage in a retrieval system or retransmission, in any form or by any means, electronic, mechanical or otherwise, for reasons other than personal use, is strictly prohibited without prior written permission. | |
CAS No. |
4286-55-9 | |
Record name | 6-Bromo-1-hexanol | |
Source | CAS Common Chemistry | |
URL | https://commonchemistry.cas.org/detail?cas_rn=4286-55-9 | |
Description | CAS Common Chemistry is an open community resource for accessing chemical information. Nearly 500,000 chemical substances from CAS REGISTRY cover areas of community interest, including common and frequently regulated chemicals, and those relevant to high school and undergraduate chemistry classes. This chemical information, curated by our expert scientists, is provided in alignment with our mission as a division of the American Chemical Society. | |
Explanation | The data from CAS Common Chemistry is provided under a CC-BY-NC 4.0 license, unless otherwise stated. | |
Record name | 6-Bromo-1-hexanol | |
Source | ChemIDplus | |
URL | https://pubchem.ncbi.nlm.nih.gov/substance/?source=chemidplus&sourceid=0004286559 | |
Description | ChemIDplus is a free, web search system that provides access to the structure and nomenclature authority files used for the identification of chemical substances cited in National Library of Medicine (NLM) databases, including the TOXNET system. | |
Record name | 6-Bromo-1-hexanol | |
Source | DrugBank | |
URL | https://www.drugbank.ca/drugs/DB04637 | |
Description | The DrugBank database is a unique bioinformatics and cheminformatics resource that combines detailed drug (i.e. chemical, pharmacological and pharmaceutical) data with comprehensive drug target (i.e. sequence, structure, and pathway) information. | |
Explanation | Creative Common's Attribution-NonCommercial 4.0 International License (http://creativecommons.org/licenses/by-nc/4.0/legalcode) | |
Record name | 1-Hexanol, 6-bromo- | |
Source | EPA Chemicals under the TSCA | |
URL | https://www.epa.gov/chemicals-under-tsca | |
Description | EPA Chemicals under the Toxic Substances Control Act (TSCA) collection contains information on chemicals and their regulations under TSCA, including non-confidential content from the TSCA Chemical Substance Inventory and Chemical Data Reporting. | |
Record name | 1-Hexanol, 6-bromo- | |
Source | EPA DSSTox | |
URL | https://comptox.epa.gov/dashboard/DTXSID2063397 | |
Description | DSSTox provides a high quality public chemistry resource for supporting improved predictive toxicology. | |
Record name | 6-Bromohexan-1-ol | |
Source | European Chemicals Agency (ECHA) | |
URL | https://echa.europa.eu/substance-information/-/substanceinfo/100.126.807 | |
Description | The European Chemicals Agency (ECHA) is an agency of the European Union which is the driving force among regulatory authorities in implementing the EU's groundbreaking chemicals legislation for the benefit of human health and the environment as well as for innovation and competitiveness. | |
Explanation | Use of the information, documents and data from the ECHA website is subject to the terms and conditions of this Legal Notice, and subject to other binding limitations provided for under applicable law, the information, documents and data made available on the ECHA website may be reproduced, distributed and/or used, totally or in part, for non-commercial purposes provided that ECHA is acknowledged as the source: "Source: European Chemicals Agency, http://echa.europa.eu/". Such acknowledgement must be included in each copy of the material. ECHA permits and encourages organisations and individuals to create links to the ECHA website under the following cumulative conditions: Links can only be made to webpages that provide a link to the Legal Notice page. | |
Record name | 6-BROMO-1-HEXANOL | |
Source | FDA Global Substance Registration System (GSRS) | |
URL | https://gsrs.ncats.nih.gov/ginas/app/beta/substances/3U3A1ZLY0T | |
Description | The FDA Global Substance Registration System (GSRS) enables the efficient and accurate exchange of information on what substances are in regulated products. Instead of relying on names, which vary across regulatory domains, countries, and regions, the GSRS knowledge base makes it possible for substances to be defined by standardized, scientific descriptions. | |
Explanation | Unless otherwise noted, the contents of the FDA website (www.fda.gov), both text and graphics, are not copyrighted. They are in the public domain and may be republished, reprinted and otherwise used freely by anyone without the need to obtain permission from FDA. Credit to the U.S. Food and Drug Administration as the source is appreciated but not required. | |
Retrosynthesis Analysis
AI-Powered Synthesis Planning: Our tool employs the Template_relevance Pistachio, Template_relevance Bkms_metabolic, Template_relevance Pistachio_ringbreaker, Template_relevance Reaxys, Template_relevance Reaxys_biocatalysis model, leveraging a vast database of chemical reactions to predict feasible synthetic routes.
One-Step Synthesis Focus: Specifically designed for one-step synthesis, it provides concise and direct routes for your target compounds, streamlining the synthesis process.
Accurate Predictions: Utilizing the extensive PISTACHIO, BKMS_METABOLIC, PISTACHIO_RINGBREAKER, REAXYS, REAXYS_BIOCATALYSIS database, our tool offers high-accuracy predictions, reflecting the latest in chemical research and data.
Strategy Settings
Precursor scoring | Relevance Heuristic |
---|---|
Min. plausibility | 0.01 |
Model | Template_relevance |
Template Set | Pistachio/Bkms_metabolic/Pistachio_ringbreaker/Reaxys/Reaxys_biocatalysis |
Top-N result to add to graph | 6 |
Feasible Synthetic Routes
Disclaimer and Information on In-Vitro Research Products
Please be aware that all articles and product information presented on BenchChem are intended solely for informational purposes. The products available for purchase on BenchChem are specifically designed for in-vitro studies, which are conducted outside of living organisms. In-vitro studies, derived from the Latin term "in glass," involve experiments performed in controlled laboratory settings using cells or tissues. It is important to note that these products are not categorized as medicines or drugs, and they have not received approval from the FDA for the prevention, treatment, or cure of any medical condition, ailment, or disease. We must emphasize that any form of bodily introduction of these products into humans or animals is strictly prohibited by law. It is essential to adhere to these guidelines to ensure compliance with legal and ethical standards in research and experimentation.