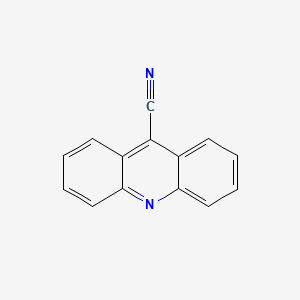
acridine-9-carbonitrile
Overview
Description
acridine-9-carbonitrile is an organic compound with the molecular formula C14H8N2. It is a derivative of acridine, characterized by the presence of a cyano group (-CN) attached to the ninth position of the acridine ring system. This compound is known for its applications in various scientific fields due to its unique chemical properties .
Mechanism of Action
Target of Action
The primary targets of acridine-9-carbonitrile are cancer cell lines . It has shown high cytotoxic activity against various types of cancer cells, including leukemia, breast cancer, and colon carcinoma cells .
Mode of Action
this compound primarily acts through DNA intercalation . This process involves the insertion of the planar acridine molecule between the base pairs of the DNA double helix . This interaction with DNA and related enzymes is principally responsible for the mode of action of acridine .
Biochemical Pathways
The intercalation of this compound into DNA can lead to significant impacts on biological processes involving DNA and related enzymes . This can affect various biochemical pathways, leading to downstream effects such as the inhibition of topoisomerase or telomerase enzymes .
Pharmacokinetics
The pharmacokinetics of this compound involves absorption, distribution, metabolism, and excretion (ADME) . The compound’s ability to penetrate the blood-brain barrier is important for the treatment of CNS tumors or neurodegenerative diseases . The pharmacokinetic profiles of acridine drugs can be improved by conjugation with various nanoobjects such as liposomes or dendrimers .
Result of Action
The result of the action of this compound is primarily observed in its potent anti-cancer activity . It has demonstrated cytotoxic activity against various cancer cell lines . For example, it has shown promising anti-tumor effects against UDP-UGT’s in leukemia cell lines .
Biochemical Analysis
Cellular Effects
The effects of 9-Acridinecarbonitrile on cellular processes are profound. It has been shown to induce cell cycle arrest and apoptosis in various cancer cell lines. The compound’s ability to intercalate into DNA disrupts the normal function of the cell, leading to the activation of cell cycle checkpoints and the initiation of programmed cell death. Additionally, 9-Acridinecarbonitrile influences cell signaling pathways, gene expression, and cellular metabolism. For instance, it can modulate the activity of transcription factors and other proteins involved in cell growth and survival .
Molecular Mechanism
At the molecular level, 9-Acridinecarbonitrile exerts its effects primarily through DNA intercalation. This interaction disrupts the normal helical structure of DNA, leading to the inhibition of DNA-dependent enzymes such as topoisomerases. The compound can also induce the formation of reactive oxygen species (ROS), which cause oxidative damage to cellular components, further contributing to its cytotoxic effects. Additionally, 9-Acridinecarbonitrile can affect gene expression by altering the binding of transcription factors to DNA, leading to changes in the expression of genes involved in cell cycle regulation and apoptosis .
Temporal Effects in Laboratory Settings
In laboratory settings, the effects of 9-Acridinecarbonitrile can change over time. The compound’s stability and degradation are critical factors that influence its long-term effects on cellular function. Studies have shown that 9-Acridinecarbonitrile can remain stable under certain conditions, but it may degrade over time, leading to a decrease in its biological activity. Long-term exposure to 9-Acridinecarbonitrile in in vitro and in vivo studies has demonstrated sustained cytotoxic effects, with potential implications for its use as a therapeutic agent .
Dosage Effects in Animal Models
The effects of 9-Acridinecarbonitrile vary with different dosages in animal models. At lower doses, the compound may exhibit minimal toxicity, while higher doses can lead to significant cytotoxic effects. Studies have shown that there is a threshold dose above which 9-Acridinecarbonitrile induces severe toxicity, including damage to vital organs and tissues. These findings highlight the importance of determining the optimal dosage for therapeutic applications to minimize adverse effects while maximizing efficacy .
Metabolic Pathways
9-Acridinecarbonitrile is involved in various metabolic pathways, interacting with enzymes and cofactors that influence its metabolism. The compound can undergo phase I and phase II metabolic reactions, including oxidation, reduction, and conjugation. These metabolic processes can affect the compound’s bioavailability, distribution, and elimination from the body. Additionally, 9-Acridinecarbonitrile can influence metabolic flux and metabolite levels, potentially altering cellular energy metabolism and other biochemical pathways .
Transport and Distribution
The transport and distribution of 9-Acridinecarbonitrile within cells and tissues are critical factors that determine its biological activity. The compound can be transported across cell membranes by specific transporters and binding proteins. Once inside the cell, 9-Acridinecarbonitrile can accumulate in various cellular compartments, including the nucleus, where it exerts its effects on DNA. The distribution of 9-Acridinecarbonitrile within tissues can also influence its therapeutic potential and toxicity .
Subcellular Localization
The subcellular localization of 9-Acridinecarbonitrile plays a crucial role in its activity and function. The compound can be targeted to specific cellular compartments, such as the nucleus, mitochondria, or endoplasmic reticulum, through various targeting signals and post-translational modifications. These localization patterns can affect the compound’s interactions with biomolecules and its overall biological activity. For example, the accumulation of 9-Acridinecarbonitrile in the nucleus can enhance its ability to intercalate into DNA and inhibit DNA-dependent enzymes .
Preparation Methods
Synthetic Routes and Reaction Conditions: The synthesis of acridine-9-carbonitrile typically involves the reaction of acridine with cyanogen bromide (BrCN) under controlled conditions. The reaction is carried out in an inert atmosphere, often using solvents like dimethylformamide (DMF) or dimethyl sulfoxide (DMSO) to facilitate the process. The reaction mixture is heated to a specific temperature to ensure the complete conversion of acridine to this compound .
Industrial Production Methods: On an industrial scale, the production of this compound follows similar synthetic routes but with optimized reaction conditions to enhance yield and purity. The use of continuous flow reactors and advanced purification techniques, such as recrystallization and chromatography, ensures the efficient production of high-purity this compound .
Chemical Reactions Analysis
Types of Reactions: acridine-9-carbonitrile undergoes various chemical reactions, including:
Oxidation: The compound can be oxidized using strong oxidizing agents like potassium permanganate (KMnO4) or chromium trioxide (CrO3), leading to the formation of acridine-9-carboxylic acid.
Reduction: Reduction of this compound can be achieved using reducing agents such as lithium aluminum hydride (LiAlH4) or sodium borohydride (NaBH4), resulting in the formation of 9-aminoacridine.
Substitution: The cyano group in this compound can be substituted with other functional groups through nucleophilic substitution reactions.
Common Reagents and Conditions:
Oxidation: Potassium permanganate (KMnO4), chromium trioxide (CrO3)
Reduction: Lithium aluminum hydride (LiAlH4), sodium borohydride (NaBH4)
Substitution: Sodium methoxide (NaOCH3), potassium tert-butoxide (KOtBu)
Major Products Formed:
Oxidation: Acridine-9-carboxylic acid
Reduction: 9-Aminoacridine
Substitution: Various substituted acridines depending on the nucleophile used.
Scientific Research Applications
acridine-9-carbonitrile has a wide range of applications in scientific research, including:
Chemistry: It is used as a precursor for the synthesis of various acridine derivatives, which are important in the development of dyes, pigments, and organic semiconductors.
Biology: The compound is studied for its potential as an intercalating agent in DNA, which makes it useful in genetic research and the development of molecular probes.
Medicine: this compound and its derivatives are investigated for their potential as anticancer agents due to their ability to interact with nucleic acids and inhibit the proliferation of cancer cells.
Comparison with Similar Compounds
9-Aminoacridine: Similar to acridine-9-carbonitrile, 9-Aminoacridine is known for its DNA intercalating properties and is used in genetic research and as an antiseptic.
Acridine Orange: This compound is a cell-permeable dye that also intercalates into DNA and is used in fluorescence microscopy and flow cytometry.
Acridine Yellow: Another dye that intercalates into DNA, used in histology and cytogenetics.
Uniqueness of this compound: What sets this compound apart from its similar compounds is its cyano group, which imparts unique electronic properties and reactivity.
Properties
IUPAC Name |
acridine-9-carbonitrile | |
---|---|---|
Source | PubChem | |
URL | https://pubchem.ncbi.nlm.nih.gov | |
Description | Data deposited in or computed by PubChem | |
InChI |
InChI=1S/C14H8N2/c15-9-12-10-5-1-3-7-13(10)16-14-8-4-2-6-11(12)14/h1-8H | |
Source | PubChem | |
URL | https://pubchem.ncbi.nlm.nih.gov | |
Description | Data deposited in or computed by PubChem | |
InChI Key |
ZBCDNEFWYPYAGB-UHFFFAOYSA-N | |
Source | PubChem | |
URL | https://pubchem.ncbi.nlm.nih.gov | |
Description | Data deposited in or computed by PubChem | |
Canonical SMILES |
C1=CC=C2C(=C1)C(=C3C=CC=CC3=N2)C#N | |
Source | PubChem | |
URL | https://pubchem.ncbi.nlm.nih.gov | |
Description | Data deposited in or computed by PubChem | |
Molecular Formula |
C14H8N2 | |
Source | PubChem | |
URL | https://pubchem.ncbi.nlm.nih.gov | |
Description | Data deposited in or computed by PubChem | |
DSSTOX Substance ID |
DTXSID80201376 | |
Record name | 9-Acridinecarbonitrile | |
Source | EPA DSSTox | |
URL | https://comptox.epa.gov/dashboard/DTXSID80201376 | |
Description | DSSTox provides a high quality public chemistry resource for supporting improved predictive toxicology. | |
Molecular Weight |
204.23 g/mol | |
Source | PubChem | |
URL | https://pubchem.ncbi.nlm.nih.gov | |
Description | Data deposited in or computed by PubChem | |
CAS No. |
5326-19-2 | |
Record name | 9-Acridinecarbonitrile | |
Source | ChemIDplus | |
URL | https://pubchem.ncbi.nlm.nih.gov/substance/?source=chemidplus&sourceid=0005326192 | |
Description | ChemIDplus is a free, web search system that provides access to the structure and nomenclature authority files used for the identification of chemical substances cited in National Library of Medicine (NLM) databases, including the TOXNET system. | |
Record name | 9-Acridinecarbonitrile | |
Source | DTP/NCI | |
URL | https://dtp.cancer.gov/dtpstandard/servlet/dwindex?searchtype=NSC&outputformat=html&searchlist=273 | |
Description | The NCI Development Therapeutics Program (DTP) provides services and resources to the academic and private-sector research communities worldwide to facilitate the discovery and development of new cancer therapeutic agents. | |
Explanation | Unless otherwise indicated, all text within NCI products is free of copyright and may be reused without our permission. Credit the National Cancer Institute as the source. | |
Record name | 9-Acridinecarbonitrile | |
Source | EPA DSSTox | |
URL | https://comptox.epa.gov/dashboard/DTXSID80201376 | |
Description | DSSTox provides a high quality public chemistry resource for supporting improved predictive toxicology. | |
Record name | acridine-9-carbonitrile | |
Source | European Chemicals Agency (ECHA) | |
URL | https://echa.europa.eu/information-on-chemicals | |
Description | The European Chemicals Agency (ECHA) is an agency of the European Union which is the driving force among regulatory authorities in implementing the EU's groundbreaking chemicals legislation for the benefit of human health and the environment as well as for innovation and competitiveness. | |
Explanation | Use of the information, documents and data from the ECHA website is subject to the terms and conditions of this Legal Notice, and subject to other binding limitations provided for under applicable law, the information, documents and data made available on the ECHA website may be reproduced, distributed and/or used, totally or in part, for non-commercial purposes provided that ECHA is acknowledged as the source: "Source: European Chemicals Agency, http://echa.europa.eu/". Such acknowledgement must be included in each copy of the material. ECHA permits and encourages organisations and individuals to create links to the ECHA website under the following cumulative conditions: Links can only be made to webpages that provide a link to the Legal Notice page. | |
Retrosynthesis Analysis
AI-Powered Synthesis Planning: Our tool employs the Template_relevance Pistachio, Template_relevance Bkms_metabolic, Template_relevance Pistachio_ringbreaker, Template_relevance Reaxys, Template_relevance Reaxys_biocatalysis model, leveraging a vast database of chemical reactions to predict feasible synthetic routes.
One-Step Synthesis Focus: Specifically designed for one-step synthesis, it provides concise and direct routes for your target compounds, streamlining the synthesis process.
Accurate Predictions: Utilizing the extensive PISTACHIO, BKMS_METABOLIC, PISTACHIO_RINGBREAKER, REAXYS, REAXYS_BIOCATALYSIS database, our tool offers high-accuracy predictions, reflecting the latest in chemical research and data.
Strategy Settings
Precursor scoring | Relevance Heuristic |
---|---|
Min. plausibility | 0.01 |
Model | Template_relevance |
Template Set | Pistachio/Bkms_metabolic/Pistachio_ringbreaker/Reaxys/Reaxys_biocatalysis |
Top-N result to add to graph | 6 |
Feasible Synthetic Routes
Disclaimer and Information on In-Vitro Research Products
Please be aware that all articles and product information presented on BenchChem are intended solely for informational purposes. The products available for purchase on BenchChem are specifically designed for in-vitro studies, which are conducted outside of living organisms. In-vitro studies, derived from the Latin term "in glass," involve experiments performed in controlled laboratory settings using cells or tissues. It is important to note that these products are not categorized as medicines or drugs, and they have not received approval from the FDA for the prevention, treatment, or cure of any medical condition, ailment, or disease. We must emphasize that any form of bodily introduction of these products into humans or animals is strictly prohibited by law. It is essential to adhere to these guidelines to ensure compliance with legal and ethical standards in research and experimentation.