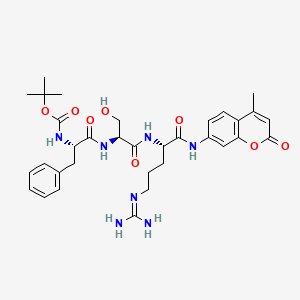
Boc-phe-ser-arg-mca
Overview
Description
The compound tertiary-Butyloxycarbonyl-phenylalanyl-seryl-arginyl-4-methylcoumarin-7-amide (Boc-phe-ser-arg-mca) is a synthetic peptide substrate used primarily in biochemical assays. It has a molecular formula of C33H43N7O8 and a molecular weight of 665.748 g/mol . This compound is often utilized in the study of proteolytic enzymes, particularly serine proteases, due to its ability to release a fluorescent product upon enzymatic cleavage .
Preparation Methods
Solid-Phase Peptide Synthesis (SPPS)
Resin Selection and Initial Attachment
The synthesis begins with the selection of a solid-phase resin, typically a polystyrene-based support functionalized with acid-labile linkers such as Wang or Rink amide resin. The first amino acid, arginine (Arg), is attached to the resin via its C-terminus using a coupling agent such as N,N'-diisopropylcarbodiimide (DIC) in the presence of Oxyma Pure as an additive to minimize racemization. The resin is pre-swollen in dimethylformamide (DMF), and the coupling reaction proceeds at 75°C for 1–2 hours to ensure >99% efficiency. The Boc (tert-butyloxycarbonyl) group protects the N-terminus of phenylalanine (Phe) during subsequent couplings.
Sequential Amino Acid Coupling
The peptide chain is elongated through iterative cycles of deprotection, coupling, and washing:
- Deprotection : The Boc group is removed using trifluoroacetic acid (TFA) in dichloromethane (DCM), followed by neutralization with N,N-diisopropylethylamine (DIPEA).
- Coupling : Subsequent amino acids (Ser, Phe) are coupled using DIC/Oxyma Pure in DMF. Each coupling step is monitored via Kaiser testing to confirm completion.
- Capping : Unreacted amino groups are acetylated using acetic anhydride (Ac₂O) and DIPEA to prevent truncated sequences.
The final residue, 4-methylcoumarin-7-amide (MCA), is introduced via amidation at the C-terminus under mild acidic conditions to preserve side-chain protections.
Cleavage and Deprotection
Resin Cleavage Conditions
The peptide-resin is treated with a cleavage cocktail comprising TFA (87.5%), triisopropylsilane (TIS, 5%), water (5%), and ethanedithiol (EDT, 2.5%) for 3 hours at room temperature. This mixture simultaneously cleaves the peptide from the resin and removes acid-labile protecting groups (e.g., Boc). The reaction is quenched by evaporating TFA under nitrogen flow, followed by precipitation in cold diethyl ether.
Side-Chain Deprotection
Boc-Phe-Ser-Arg-MCA contains side-chain protecting groups (e.g., Arg’s Pbf or Mtr groups), which are retained during resin cleavage. Final deprotection is achieved via hydrogenolysis or treatment with silver acetate (AgOAc) in DMF, followed by reduction with dithiothreitol (DTT) to eliminate disulfide byproducts.
Purification and Characterization
Reverse-Phase High-Performance Liquid Chromatography (RP-HPLC)
Crude peptide is purified using a C8 semi-preparative column with a gradient of acetonitrile (0–40%) in 0.1% aqueous TFA over 80 minutes. Key parameters include:
Parameter | Value |
---|---|
Column | XBridge® C8, 300 Å |
Flow Rate | 35 mL/min |
Detection | UV at 214 nm |
Eluent | 0.1% TFA in H₂O/CH₃CN |
Fractions containing the target compound are lyophilized to yield a white solid.
Mass Spectrometry (MS) and Purity Assessment
Electrospray ionization mass spectrometry (ESI-MS) confirms molecular weight (m/z = 937.95 [M+2H]²⁺). Analytical HPLC with a C18 column ensures >95% purity, critical for enzymatic assays.
Cyclization and Stability Optimization
Thioether Cyclization
For stabilized analogues, cyclization is performed in DMSO or acetonitrile/water (1:1) with DIPEA to facilitate intramolecular thioether bond formation. Reaction progress is monitored via LC-MS, with typical completion within 1 hour.
Industrial-Scale Production
Automation and Process Intensification
Large-scale synthesis employs automated peptide synthesizers with in-line HPLC monitoring. Multi-kilogram batches achieve coupling efficiencies of >98% through optimized reagent stoichiometry (3:1 excess of amino acids).
Cost and Yield Considerations
Industrial yields range from 10–15% after purification, driven by losses during cyclization and HPLC steps. Scalable alternatives, such as continuous-flow reactors, are under investigation to improve throughput.
Case Study: Synthesis of a Functional Analog
A recent protocol for a related peptide, Boc-Leu-Ser-Thr-Arg-MCA, highlights the impact of residue substitution on synthesis efficiency. Replacing Phe with Leu reduced coupling times by 20% due to improved solubility in DMF, underscoring the role of side-chain chemistry in SPPS optimization.
Chemical Reactions Analysis
Enzymatic Hydrolysis and Protease Interactions
Boc-phe-ser-arg-mca undergoes proteolytic cleavage at the arginine residue by serine and cysteine proteases, releasing the fluorescent 7-amino-4-methylcoumarin (AMC) moiety. This reaction serves as the basis for quantifying enzyme activity in biochemical assays .
Key Enzymes and Specificity
-
Trypsin and Tryptase : Cleave the substrate at the C-terminal arginine, producing AMC fluorescence .
-
Arg-Gingipain : A cysteine protease from Porphyromonas gingivalis that hydrolyzes the substrate with high specificity.
-
ME-2 (Snake Venom Enzyme) : Hydrolyzes this compound, albeit with lower efficiency compared to other substrates like Pro-Phe-Arg-MCA .
Table 1: Kinetic Parameters for Enzymatic Hydrolysis
Enzyme | Substrate | (μM) | Inhibition Profile |
---|---|---|---|
Trypsin | This compound | 30.1 ± 3.8 | Diisopropyl fluorophosphate |
Arg-Gingipain | This compound | 17.9 ± 1.6 | Leupeptin (strong inhibition) |
ME-2 | This compound | Not reported | Aprotinin, E-64 (weak) |
Data derived from enzyme kinetics studies .
Solvent Interactions and Solubility
The compound’s reactivity is influenced by solvent composition:
-
DMSO Effects : Higher DMSO concentrations (10% vs. 2%) reduce hydrolysis rates by 30–50% due to substrate aggregation and enzyme inhibition .
-
Precipitation Issues : this compound forms precipitates in low-DMSO buffers (<2%), necessitating optimized solvent systems for assays .
Table 2: Solvent Impact on Hydrolysis Rates
Substrate | DMSO (%) | Hydrolysis Rate (nmol/L/min) |
---|---|---|
This compound | 2 | 50.1 ± 3.9 |
This compound | 10 | 38.0 ± 5.6 |
Data from kinetic assays in seawater and microbial studies .
Chemical Modifications and Conjugation
This compound is chemically modified for advanced applications:
-
Fluorescent Probes : Conjugation with AMC enables real-time tracking of protease activity in live cells .
-
Drug Development : Incorporation into peptide inhibitors targeting thrombin and factor XIa, with IC values in the nanomolar range .
Mechanistic Insight :
The compound’s tert-butyloxycarbonyl (Boc) group enhances stability during synthesis, while the AMC fluorophore allows sensitive detection of enzymatic cleavage .
Scientific Research Applications
Enzyme Assays
Boc-phe-ser-arg-mca serves as a substrate for measuring the activity of serine proteases. Upon cleavage by these enzymes, it releases a fluorescent group, 7-amino-4-methylcoumarin (AMC), which can be quantified using fluorescence spectroscopy. This method allows researchers to assess the enzymatic activity in various biological samples and evaluate the efficacy of protease inhibitors.
Table 1: Summary of Enzyme Activity Measurement
Enzyme | Substrate | Fluorescent Product | Detection Method |
---|---|---|---|
Trypsin | This compound | 7-amino-4-methylcoumarin | Fluorescence Spectroscopy |
Tryptase | This compound | 7-amino-4-methylcoumarin | Fluorescence Spectroscopy |
Kallikreins | This compound | 7-amino-4-methylcoumarin | Fluorescence Spectroscopy |
Biochemical Research
The compound is extensively utilized in biochemical assays to study enzyme kinetics and inhibition. It has been demonstrated that this compound interacts effectively with trypsin-like serine proteases, providing insights into enzyme-substrate interactions and specificity.
Case Study: Inhibition Studies
Inhibition studies have shown that this compound can be hydrolyzed by serine proteases present in human skin, highlighting its role in physiological processes such as desquamation. The hydrolytic activity was inhibited by serine protease inhibitors like diisopropyl fluorophosphate and aprotinin, indicating its potential use in studying skin-related enzymatic functions .
Drug Development
This compound is also pivotal in pharmaceutical research for designing new drugs targeting specific enzymes or receptors. Its structure allows for easy incorporation into peptide chains, enhancing the synthesis of therapeutic agents.
Applications in Drug Design
- Targeting Serine Proteases: The compound's ability to selectively interact with serine proteases makes it an ideal candidate for developing drugs aimed at modulating these enzymes' activities.
- Fluorescent Probes: Its fluorescent properties enable the creation of probes that can visualize cellular activities, aiding in drug screening and development processes .
Protein Engineering
In protein engineering, this compound can be incorporated into proteins to modify their function or stability. This application is significant for developing more effective biocatalysts or therapeutic proteins.
Cellular Effects and Mechanisms
This compound influences various cellular processes by serving as a substrate for proteases involved in cell signaling and metabolism. The molecular mechanism involves its binding to the active site of serine proteases, leading to the cleavage of the peptide bond at the arginine residue and subsequent release of the fluorescent AMC moiety .
Table 2: Summary of Cellular Interactions
Cellular Process | Mechanism |
---|---|
Inflammation | Cleavage by trypsin and tryptase |
Tissue Remodeling | Interaction with kallikreins |
Immune Response | Substrate for serine proteases |
Mechanism of Action
The mechanism of action of tertiary-Butyloxycarbonyl-phenylalanyl-seryl-arginyl-4-methylcoumarin-7-amide involves its recognition and cleavage by serine proteases. These enzymes bind to the peptide substrate and catalyze the hydrolysis of the peptide bond, resulting in the release of the fluorescent product. The molecular targets include the active sites of serine proteases, where the catalytic triad (serine, histidine, and aspartate) facilitates the cleavage reaction .
Comparison with Similar Compounds
Similar Compounds
Z-Phe-Arg-MCA: Another peptide substrate used for trypsin-like proteases.
Suc-Leu-Leu-Val-Tyr-MCA: A substrate for chymotrypsin.
Boc-Val-Pro-Arg-MCA: Used for studying thrombin activity.
Uniqueness
Tertiary-Butyloxycarbonyl-phenylalanyl-seryl-arginyl-4-methylcoumarin-7-amide is unique due to its specific sequence, which makes it an ideal substrate for certain serine proteases. Its ability to release a highly fluorescent product upon cleavage allows for sensitive and accurate detection of enzyme activity .
Biological Activity
Boc-Phe-Ser-Arg-MCA (Boc = tert-butyloxycarbonyl, MCA = 4-methylcoumaryl-7-amide) is a synthetic peptide substrate widely used in biochemical research to study proteolytic enzymes. This compound serves as a substrate for various proteases, particularly cysteine proteases, and its hydrolysis can be indicative of enzyme activity. The biological activity of this compound has been explored in various contexts, including plant biotechnology and microbiological studies.
1. Proteolytic Activity and Enzyme Specificity
This compound has been shown to be hydrolyzed by specific proteases, indicating its utility in enzyme assays. Notably, research has identified that the hydrolysis of this compound is inhibited by leupeptin, a known cysteine protease inhibitor, while showing weak inhibition by E-64. This suggests that the enzyme responsible for hydrolyzing this compound is likely a cysteine protease with an alkaline pH optimum .
Table 1: Enzyme Inhibition Profiles for this compound
Inhibitor | Inhibition Strength |
---|---|
Leupeptin | Strong |
E-64 | Weak |
Pepstatin A | None |
1,10-Phenanthroline | None |
PMSF | None |
2. Application in Plant Biotechnology
Research into the differentiation of tracheary elements (TE) in Zinnia cells has demonstrated that this compound can serve as a substrate to study endopeptidase activities induced during this process. The hydrolyzing activity was observed to peak during specific culture conditions conducive to TE differentiation, highlighting the role of this peptide in understanding plant developmental biology .
3. Microbial Studies
In microbial contexts, this compound has been utilized to assess extracellular aminopeptidase production among bacterial strains. The results indicated that while some strains exhibited significant aminopeptidase activity, the hydrolysis of Boc-Phe-Ser-Thr-Arg-MCA was notably weak, suggesting varying levels of proteolytic activity among different microbial species .
Case Study 1: Zinnia Cell Differentiation
A study investigated the enzymatic activities associated with TE differentiation using this compound as a substrate. The findings revealed that the hydrolyzing activity was significantly induced under differentiation-inductive conditions, suggesting its potential role as a marker for protease activity during plant development .
Case Study 2: Bacterial Aminopeptidase Production
In another study focused on marine bacteria isolated from the Seto Inland Sea, researchers assessed the production of extracellular aminopeptidases using various MCA substrates including this compound. The results indicated distinct profiles of enzymatic activity that could be correlated with nutrient availability and bacterial growth conditions .
5. Conclusion
This compound serves as an important tool in biochemical research for studying proteolytic enzymes across different biological systems. Its specificity for cysteine proteases and its application in both plant and microbial studies highlight its versatility and significance in understanding enzymatic processes.
Q & A
Basic Questions
Q. What are the standard protocols for preparing Boc-Phe-Ser-Arg-MCA solutions in enzymatic assays?
this compound exhibits solubility challenges in aqueous solutions, particularly at lower DMSO concentrations (e.g., 1–2% DMSO). To mitigate precipitation, prepare stock solutions in 10% DMSO and dilute with assay buffers to maintain substrate solubility while minimizing solvent interference. Pre-filter solutions to remove aggregates before use. Ensure substrate stability by avoiding prolonged storage in diluted forms .
Q. How is this compound used to measure trypsin-like protease activity?
this compound is cleaved by trypsin-like proteases at the Arg-MCA bond, releasing fluorescent 4-methylcoumaryl-7-amide (MCA). Standard protocols involve incubating the substrate with enzyme extracts, monitoring fluorescence intensity (excitation 380 nm, emission 460 nm) over time. Calibrate assays using known enzyme concentrations and account for background fluorescence from non-enzymatic hydrolysis. This substrate has been validated in UV-irradiated skin models to study tryptase activity .
Q. What solubility challenges are associated with this compound in enzymatic assays?
this compound forms precipitates in low-DMSO conditions (e.g., 1–2% DMSO), requiring higher solvent concentrations (≥10% DMSO) for solubility. However, elevated DMSO may inhibit enzyme activity or alter kinetic parameters. To balance solubility and assay validity, optimize DMSO concentrations using pilot experiments and validate hydrolysis rates under varying solvent conditions .
Advanced Research Questions
Q. How does DMSO concentration affect this compound hydrolysis rates in enzyme kinetics studies?
DMSO acts as a competitive inhibitor for dissolved trypsin-type enzymes at higher concentrations (e.g., 10% DMSO), reducing hydrolysis rates by altering substrate-enzyme binding. For example, in seawater assays, 10% DMSO suppressed aminopeptidase and chymotrypsin-type activities but showed variable effects on trypsin-like proteases depending on substrate concentration. Use Michaelis-Menten kinetics to model solvent effects and distinguish between enzyme inhibition and substrate solubility limitations .
Q. How can researchers design experiments to address contradictory hydrolysis data at varying substrate concentrations?
Contradictory results (e.g., higher hydrolysis rates in 2% vs. 10% DMSO at low substrate concentrations but reversed trends at high concentrations) may arise from solubility-driven artifacts or solvent-enzyme interactions. To resolve discrepancies:
- Perform solubility tests across DMSO concentrations.
- Conduct control experiments with structurally similar substrates.
- Use Lineweaver-Burk plots to differentiate competitive vs. non-competitive inhibition mechanisms .
Q. What methodological considerations are critical when optimizing this compound-based assays for novel proteases?
Key considerations include:
- Substrate specificity : Validate cleavage specificity using protease inhibitors (e.g., aprotinin for trypsin-like enzymes).
- Kinetic parameters : Calculate and under standardized solvent conditions.
- Interference checks : Test for fluorescence quenching by assay components (e.g., metal ions).
- Reproducibility : Document DMSO lot variability and pre-incubation times to ensure consistency .
Q. How can researchers validate this compound as a substrate in novel protease research?
Validation steps include:
- Enzyme specificity : Compare hydrolysis rates with known substrates (e.g., Z-Phe-Arg-MCA for trypsin).
- Inhibition assays : Confirm activity suppression using class-specific inhibitors.
- Cross-validation : Correlate fluorescence data with alternative methods (e.g., HPLC).
- Biological relevance : Test in physiologically relevant models (e.g., UV-irradiated skin for tryptase studies) .
Properties
IUPAC Name |
tert-butyl N-[(2S)-1-[[(2S)-1-[[(2S)-5-(diaminomethylideneamino)-1-[(4-methyl-2-oxochromen-7-yl)amino]-1-oxopentan-2-yl]amino]-3-hydroxy-1-oxopropan-2-yl]amino]-1-oxo-3-phenylpropan-2-yl]carbamate | |
---|---|---|
Source | PubChem | |
URL | https://pubchem.ncbi.nlm.nih.gov | |
Description | Data deposited in or computed by PubChem | |
InChI |
InChI=1S/C33H43N7O8/c1-19-15-27(42)47-26-17-21(12-13-22(19)26)37-28(43)23(11-8-14-36-31(34)35)38-30(45)25(18-41)39-29(44)24(16-20-9-6-5-7-10-20)40-32(46)48-33(2,3)4/h5-7,9-10,12-13,15,17,23-25,41H,8,11,14,16,18H2,1-4H3,(H,37,43)(H,38,45)(H,39,44)(H,40,46)(H4,34,35,36)/t23-,24-,25-/m0/s1 | |
Source | PubChem | |
URL | https://pubchem.ncbi.nlm.nih.gov | |
Description | Data deposited in or computed by PubChem | |
InChI Key |
JLKJMNJZJBEYLQ-SDHOMARFSA-N | |
Source | PubChem | |
URL | https://pubchem.ncbi.nlm.nih.gov | |
Description | Data deposited in or computed by PubChem | |
Canonical SMILES |
CC1=CC(=O)OC2=C1C=CC(=C2)NC(=O)C(CCCN=C(N)N)NC(=O)C(CO)NC(=O)C(CC3=CC=CC=C3)NC(=O)OC(C)(C)C | |
Source | PubChem | |
URL | https://pubchem.ncbi.nlm.nih.gov | |
Description | Data deposited in or computed by PubChem | |
Isomeric SMILES |
CC1=CC(=O)OC2=C1C=CC(=C2)NC(=O)[C@H](CCCN=C(N)N)NC(=O)[C@H](CO)NC(=O)[C@H](CC3=CC=CC=C3)NC(=O)OC(C)(C)C | |
Source | PubChem | |
URL | https://pubchem.ncbi.nlm.nih.gov | |
Description | Data deposited in or computed by PubChem | |
Molecular Formula |
C33H43N7O8 | |
Source | PubChem | |
URL | https://pubchem.ncbi.nlm.nih.gov | |
Description | Data deposited in or computed by PubChem | |
DSSTOX Substance ID |
DTXSID80994332 | |
Record name | 6-Benzyl-12-(3-carbamimidamidopropyl)-4,7,10-trihydroxy-9-(hydroxymethyl)-2,2-dimethyl-N-(4-methyl-2-oxo-2H-1-benzopyran-7-yl)-3-oxa-5,8,11-triazatrideca-4,7,10-trien-13-imidic acid | |
Source | EPA DSSTox | |
URL | https://comptox.epa.gov/dashboard/DTXSID80994332 | |
Description | DSSTox provides a high quality public chemistry resource for supporting improved predictive toxicology. | |
Molecular Weight |
665.7 g/mol | |
Source | PubChem | |
URL | https://pubchem.ncbi.nlm.nih.gov | |
Description | Data deposited in or computed by PubChem | |
CAS No. |
73554-90-2 | |
Record name | tertiary-Butyloxycarbonyl-phenylalanyl-seryl-arginyl-4-methylcoumarin-7-amide | |
Source | ChemIDplus | |
URL | https://pubchem.ncbi.nlm.nih.gov/substance/?source=chemidplus&sourceid=0073554902 | |
Description | ChemIDplus is a free, web search system that provides access to the structure and nomenclature authority files used for the identification of chemical substances cited in National Library of Medicine (NLM) databases, including the TOXNET system. | |
Record name | 6-Benzyl-12-(3-carbamimidamidopropyl)-4,7,10-trihydroxy-9-(hydroxymethyl)-2,2-dimethyl-N-(4-methyl-2-oxo-2H-1-benzopyran-7-yl)-3-oxa-5,8,11-triazatrideca-4,7,10-trien-13-imidic acid | |
Source | EPA DSSTox | |
URL | https://comptox.epa.gov/dashboard/DTXSID80994332 | |
Description | DSSTox provides a high quality public chemistry resource for supporting improved predictive toxicology. | |
Retrosynthesis Analysis
AI-Powered Synthesis Planning: Our tool employs the Template_relevance Pistachio, Template_relevance Bkms_metabolic, Template_relevance Pistachio_ringbreaker, Template_relevance Reaxys, Template_relevance Reaxys_biocatalysis model, leveraging a vast database of chemical reactions to predict feasible synthetic routes.
One-Step Synthesis Focus: Specifically designed for one-step synthesis, it provides concise and direct routes for your target compounds, streamlining the synthesis process.
Accurate Predictions: Utilizing the extensive PISTACHIO, BKMS_METABOLIC, PISTACHIO_RINGBREAKER, REAXYS, REAXYS_BIOCATALYSIS database, our tool offers high-accuracy predictions, reflecting the latest in chemical research and data.
Strategy Settings
Precursor scoring | Relevance Heuristic |
---|---|
Min. plausibility | 0.01 |
Model | Template_relevance |
Template Set | Pistachio/Bkms_metabolic/Pistachio_ringbreaker/Reaxys/Reaxys_biocatalysis |
Top-N result to add to graph | 6 |
Feasible Synthetic Routes
Disclaimer and Information on In-Vitro Research Products
Please be aware that all articles and product information presented on BenchChem are intended solely for informational purposes. The products available for purchase on BenchChem are specifically designed for in-vitro studies, which are conducted outside of living organisms. In-vitro studies, derived from the Latin term "in glass," involve experiments performed in controlled laboratory settings using cells or tissues. It is important to note that these products are not categorized as medicines or drugs, and they have not received approval from the FDA for the prevention, treatment, or cure of any medical condition, ailment, or disease. We must emphasize that any form of bodily introduction of these products into humans or animals is strictly prohibited by law. It is essential to adhere to these guidelines to ensure compliance with legal and ethical standards in research and experimentation.