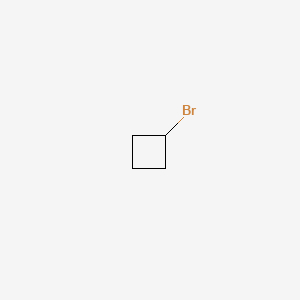
Bromocyclobutane
Overview
Description
Bromocyclobutane, also known as cyclobutyl bromide, is an organic compound with the molecular formula C4H7Br. It is a colorless liquid at room temperature and pressure, characterized by its four-membered ring structure which imparts significant ring strain. This strain makes this compound a reactive compound, often used in organic synthesis and various chemical transformations .
Preparation Methods
Direct Bromination of Cyclobutanol
The most straightforward route to bromocyclobutane involves the bromination of cyclobutanol using hydrobromic acid (HBr). This method leverages the nucleophilic substitution (SN2) mechanism, where the hydroxyl group of cyclobutanol is replaced by bromide. A patented approach optimized this reaction by employing 48% HBr at 40–50°C for 4 hours, achieving a 59% yield of crude this compound. However, this method produces significant byproducts, including 4-bromo-1-butene (23%) and cyclopropylmethyl bromide (8%), due to competing ring-opening and rearrangement pathways.
Key variables influencing yield and selectivity include:
- HBr concentration : Lower concentrations (30–60%) minimize side reactions while maintaining reactivity.
- Temperature : Elevated temperatures (>80°C) accelerate ring-opening, favoring 4-bromo-1-butene formation.
- Molar ratio : A 1:1.06 ratio of cyclobutanol to HBr balances conversion and cost.
Despite its simplicity, this method requires multi-step purification to isolate this compound from byproducts, limiting its industrial appeal.
Ring-Opening of Cyclopropylcarbinol
An innovative alternative utilizes cyclopropylcarbinol as the starting material, exploiting its strain-driven ring-opening upon bromination. When treated with HBr, the cyclopropane ring expands to form this compound via a concerted mechanism. This method, detailed in patent CN101209953A, achieves higher regioselectivity compared to cyclobutanol bromination.
Reaction Conditions and Optimization
The patent outlines the following optimized parameters:
Parameter | Range | Optimized Value |
---|---|---|
HBr concentration | 20–80% | 48% |
Temperature | 35–120°C | 40–50°C |
Reaction time | 2–8 hours | 4 hours |
Molar ratio (cyclopropylcarbinol:HBr) | 1:1–2 | 1:1.06 |
Under these conditions, the reaction produces a mixture containing 69% this compound , 23% 4-bromo-1-butene, and 8% cyclopropylmethyl bromide. The crude product requires purification via two sequential steps:
- Treatment with N-bromosuccinimide (NBS) to scavenge 4-bromo-1-butene.
- Distillation with high-boiling amines (e.g., dibenzylamine) to remove cyclopropylmethyl bromide.
This dual purification strategy elevates final purity to >98.5% with an overall yield of 13–15%.
Byproduct Formation and Mitigation
The formation of 4-bromo-1-butene and cyclopropylmethyl bromide remains a critical challenge. Computational studies suggest that 4-bromo-1-butene arises from a carbocation rearrangement pathway, while cyclopropylmethyl bromide forms via competing ring-opening of cyclopropane. The patent addresses this by:
- Lowering reaction temperatures to 40–50°C, reducing carbocation mobility.
- Using excess HBr (1.06 equivalents) to drive the equilibrium toward this compound.
- Adding radical scavengers (e.g., NBS) to terminate alkene-forming side reactions.
Industrial-Scale Purification Techniques
NBS-Mediated Purification
Crude this compound is stirred with N-bromosuccinimide (0.5 equivalents) at room temperature for 12–24 hours. This step selectively reacts with 4-bromo-1-butene via allylic bromination, converting it into non-volatile derivatives that are removed during distillation. Post-treatment, the this compound content increases from 69% to 92%.
Amine-Assisted Distillation
The semi-purified product is heated with dibenzylamine (0.2 equivalents) at 65–80°C for 48 hours. The amine coordinates with cyclopropylmethyl bromide, facilitating its decomposition into volatile byproducts. Subsequent distillation yields this compound with >99% purity.
Comparative Analysis of Methods
Method | Starting Material | Yield (Overall) | Purity | Cost Efficiency |
---|---|---|---|---|
Cyclobutanol bromination | Cyclobutanol | 40–50% | 85–90% | Moderate |
Cyclopropylcarbinol route | Cyclopropylcarbinol | 13–15% | >98.5% | High |
While the cyclopropylcarbinol method offers superior purity, its lower yield stems from multi-step purification. Recent advances in continuous-flow reactors may enhance throughput by minimizing intermediate isolation.
Chemical Reactions Analysis
Types of Reactions: Bromocyclobutane undergoes various types of chemical reactions, including:
Substitution Reactions: this compound can participate in nucleophilic substitution reactions where the bromine atom is replaced by other nucleophiles.
Elimination Reactions: It can undergo dehydrohalogenation to form cyclobutene.
Addition Reactions: this compound can react with electrophiles to form addition products.
Common Reagents and Conditions:
Nucleophiles: Such as hydroxide ions, alkoxide ions, and amines.
Bases: For elimination reactions, common bases include potassium tert-butoxide and sodium hydride.
Electrophiles: Such as bromine and chlorine for addition reactions.
Major Products:
Cyclobutene: Formed through elimination reactions.
Substituted Cyclobutanes: Formed through nucleophilic substitution reactions
Scientific Research Applications
Organic Synthesis
Bromocyclobutane serves as a versatile building block in organic synthesis. Its reactivity allows it to participate in various chemical reactions, making it useful for constructing complex molecular architectures.
- Alkylation Reactions : this compound can undergo nucleophilic substitution reactions, where the bromine atom is replaced by a nucleophile. This property facilitates the formation of larger carbon frameworks, which are essential in the synthesis of pharmaceuticals and agrochemicals.
- Cycloaddition Reactions : The compound can also participate in cycloaddition reactions, such as the Diels-Alder reaction, allowing for the formation of new cyclic structures. This is particularly valuable in synthesizing natural products and biologically active compounds.
Medicinal Chemistry
In medicinal chemistry, this compound derivatives have been investigated for their potential therapeutic applications.
- Anticancer Agents : Some studies have explored the use of this compound derivatives as anticancer agents. The presence of the bromine atom can enhance the biological activity of these compounds by improving their interaction with biological targets.
- Antimicrobial Properties : Research has indicated that certain this compound derivatives exhibit antimicrobial activity, making them candidates for developing new antibiotics. Their mechanism often involves disrupting bacterial cell membranes or inhibiting essential metabolic pathways.
Materials Science
This compound's unique properties make it suitable for various applications in materials science.
- Polymer Chemistry : this compound can be used in the synthesis of polymers through radical polymerization processes. Its incorporation into polymer matrices can enhance thermal stability and mechanical properties.
- Nanotechnology : The compound has potential applications in nanotechnology, particularly in the development of nanomaterials for drug delivery systems. Its ability to form stable complexes with various nanoparticles can improve the bioavailability and efficacy of therapeutic agents.
Case Study 1: Synthesis of this compound Derivatives
A recent study demonstrated the synthesis of novel this compound derivatives through a two-step reaction involving cyclopentadiene and elemental bromine. The resulting compounds were evaluated for their anticancer activity against various cancer cell lines. The findings indicated that certain derivatives exhibited significant cytotoxic effects, suggesting their potential as lead compounds for further development in cancer therapy.
Case Study 2: Application in Polymer Science
Another study focused on incorporating this compound into a polymer matrix to enhance its mechanical properties. The research showed that adding this compound improved the tensile strength and thermal stability of the polymer, making it suitable for applications in high-performance materials.
Mechanism of Action
The mechanism of action of bromocyclobutane involves its high ring strain, which makes it highly reactive. This reactivity allows it to participate in various chemical transformations, such as nucleophilic substitution and elimination reactions. The molecular targets and pathways involved depend on the specific reaction and the reagents used .
Comparison with Similar Compounds
Cyclobutane: A four-membered ring compound without the bromine substituent.
Bicyclobutane: A compound with two fused four-membered rings, exhibiting even higher ring strain.
Cyclopropyl Bromide: A three-membered ring compound with a bromine substituent.
Uniqueness: Bromocyclobutane is unique due to its combination of a four-membered ring structure and a bromine substituent, which imparts significant ring strain and reactivity. This makes it a valuable intermediate in organic synthesis and various chemical transformations .
Biological Activity
Bromocyclobutane is a halogenated cyclic compound that has garnered attention for its diverse biological activities. This article explores the compound's properties, mechanisms of action, and relevant case studies, highlighting its potential applications in medicinal chemistry and pharmacology.
Chemical Structure and Properties
This compound is characterized by a four-membered ring structure containing a bromine atom. The presence of halogens, particularly bromine, influences its reactivity and biological interactions. The compound's lipophilicity and molecular size contribute to its ability to penetrate biological membranes, which is crucial for its biological activity.
Antimicrobial Activity
Research indicates that this compound derivatives exhibit significant antimicrobial properties. A study evaluated various halogenated compounds against strains such as Staphylococcus aureus and Enterococcus faecalis. The results demonstrated that compounds with bromine showed enhanced antibacterial activity compared to their non-halogenated counterparts. Specifically, the minimum inhibitory concentrations (MICs) for brominated compounds ranged from 0.070 to 8.95 μM against S. aureus .
Table 1: Antimicrobial Activity of this compound Derivatives
Compound | MIC against S. aureus (μM) | MIC against E. faecalis (μM) |
---|---|---|
This compound | 0.070 - 8.95 | 4.66 - 35.8 |
Non-brominated analog | Not effective | Not effective |
Cytotoxicity
The cytotoxic effects of this compound have been assessed using various human cell lines, including THP-1 monocytic leukemia cells. The IC50 values for these compounds were found to range from 1.4 to >10 µM, indicating a dose-dependent relationship between lipophilicity and cytotoxicity . This suggests that modifications to the this compound structure can enhance its therapeutic potential while minimizing toxicity.
The biological activity of this compound is closely related to its ability to interact with cellular targets:
- Membrane Disruption : Brominated compounds can integrate into lipid membranes, altering their fluidity and permeability, which may lead to cell lysis.
- Enzyme Inhibition : Some studies suggest that this compound derivatives may inhibit key enzymes involved in bacterial metabolism, thus exerting their antimicrobial effects.
- Reactive Oxygen Species (ROS) Generation : The presence of halogens can promote oxidative stress in cells, leading to apoptosis in susceptible cell lines.
Case Studies
A notable case study involved the synthesis and evaluation of various this compound derivatives for their antimicrobial properties. The study highlighted the importance of structural modifications in enhancing biological activity:
- Case Study 1 : A series of this compound derivatives were synthesized and tested against MRSA strains. Results indicated that specific substitutions on the cyclobutane ring significantly improved antibacterial efficacy.
- Case Study 2 : In vitro studies demonstrated that certain brominated compounds exhibited selective cytotoxicity towards cancer cells while sparing normal cells, suggesting potential applications in targeted cancer therapy.
Q & A
Basic Research Questions
Q. What are the established synthetic routes for bromocyclobutane, and how do reaction conditions influence yield and purity?
this compound (C₄H₇Br, CAS 4399-47-7) is typically synthesized via bromination of cyclobutane derivatives or ring-closing reactions of brominated precursors. Key methods include:
- Radical bromination : Cyclobutane reacts with bromine under UV light, though steric strain in the cyclobutane ring may lead to side products .
- Nucleophilic substitution : Cyclobutanol derivatives treated with HBr or PBr₃, requiring precise temperature control to minimize ring-opening side reactions . Yield optimization involves GC-MS purity checks (>97% as per commercial standards) and column chromatography for isolation .
Q. Which spectroscopic and chromatographic techniques are most reliable for characterizing this compound?
- NMR spectroscopy : ¹H NMR reveals deshielded protons adjacent to bromine (δ ~4.5–5.5 ppm), while ¹³C NMR identifies carbons bonded to Br (δ ~30–40 ppm) .
- IR spectroscopy : C-Br stretching vibrations appear at 500–600 cm⁻¹ .
- GC-MS : Retention time and fragmentation patterns (e.g., m/z 135 for molecular ion) confirm identity and purity . Cross-referencing with literature databases (e.g., Beilstein) is critical for validation .
Q. How does this compound’s ring strain affect its physical properties and reactivity compared to larger cycloalkanes?
The cyclobutane ring’s high angle strain (≈90° bond angles) increases reactivity:
- Boiling point : Lower than cyclohexane derivatives due to reduced van der Waals interactions .
- Thermal stability : Prone to ring-opening reactions above 100°C, necessitating low-temperature storage . Comparative studies with bromocyclohexane can isolate strain effects .
Advanced Research Questions
Q. What mechanistic insights explain this compound’s behavior in transition-metal-catalyzed cross-coupling reactions?
this compound’s strained ring complicates oxidative addition in palladium-catalyzed reactions. Key considerations:
- Steric effects : Smaller ligands (e.g., Pd(PPh₃)₄) improve catalytic activity .
- Ring-opening pathways : Competing β-hydrogen elimination may occur, requiring deuterium labeling or computational modeling (DFT) to track intermediates . Contrast with bromocyclohexane reactivity to identify strain-induced pathways .
Q. How can contradictions in reported thermodynamic data (e.g., enthalpy of formation) for this compound be resolved?
Discrepancies arise from differing experimental setups:
- Calorimetry : Bomb calorimetry under inert atmospheres reduces decomposition artifacts .
- Computational validation : Compare experimental ΔHf values with DFT-calculated data (e.g., B3LYP/6-31G*) to identify systematic errors . Meta-analyses of historical datasets should account for purity thresholds (e.g., >97% GC) .
Q. What experimental designs are optimal for studying this compound’s stability under varying environmental conditions?
- Controlled degradation studies : Expose samples to UV light, humidity, or oxygen while monitoring decomposition via HPLC .
- Kinetic analysis : Arrhenius plots quantify degradation rates at 25–100°C, with activation energy calculations to predict shelf life .
- Structural elucidation of byproducts : Use LC-MS or X-ray crystallography to identify ring-opened products (e.g., butenyl bromides) .
Q. How do isotopic labeling strategies (e.g., ¹³C, D) enhance mechanistic studies of this compound reactions?
- Tracer studies : ¹³C-labeled this compound clarifies bond-breaking/forming steps in ring-opening reactions via NMR .
- Kinetic isotope effects (KIE) : Deuterium at reactive positions (e.g., β-hydrogens) differentiates concerted vs. stepwise mechanisms . Synthesize labeled analogs via modified Grignard or halogen-exchange protocols .
Q. Methodological Guidance
Q. What strategies mitigate challenges in reproducing this compound syntheses across laboratories?
- Standardized protocols : Document exact stoichiometry, solvent grades, and quenching methods .
- Purity validation : Require GC-MS/NMR data with baseline resolution for key impurities .
- Collaborative validation : Cross-lab replication studies identify equipment-specific variables (e.g., stirrer speed affecting mixing efficiency) .
Q. How should researchers address conflicting literature reports on this compound’s spectroscopic signatures?
- Reference materials : Use commercially available high-purity samples (e.g., >97% from Kanto Reagents) as benchmarks .
- Solvent effects : Replicate experiments in deuterated vs. non-deuterated solvents to isolate shifts .
- Machine calibration : Regular NMR magnet shimming and GC column conditioning ensure reproducibility .
Q. What computational models best predict this compound’s reactivity in novel reaction systems?
Properties
IUPAC Name |
bromocyclobutane | |
---|---|---|
Source | PubChem | |
URL | https://pubchem.ncbi.nlm.nih.gov | |
Description | Data deposited in or computed by PubChem | |
InChI |
InChI=1S/C4H7Br/c5-4-2-1-3-4/h4H,1-3H2 | |
Source | PubChem | |
URL | https://pubchem.ncbi.nlm.nih.gov | |
Description | Data deposited in or computed by PubChem | |
InChI Key |
KXVUSQIDCZRUKF-UHFFFAOYSA-N | |
Source | PubChem | |
URL | https://pubchem.ncbi.nlm.nih.gov | |
Description | Data deposited in or computed by PubChem | |
Canonical SMILES |
C1CC(C1)Br | |
Source | PubChem | |
URL | https://pubchem.ncbi.nlm.nih.gov | |
Description | Data deposited in or computed by PubChem | |
Molecular Formula |
C4H7Br | |
Source | PubChem | |
URL | https://pubchem.ncbi.nlm.nih.gov | |
Description | Data deposited in or computed by PubChem | |
DSSTOX Substance ID |
DTXSID20196010 | |
Record name | Bromocyclobutane | |
Source | EPA DSSTox | |
URL | https://comptox.epa.gov/dashboard/DTXSID20196010 | |
Description | DSSTox provides a high quality public chemistry resource for supporting improved predictive toxicology. | |
Molecular Weight |
135.00 g/mol | |
Source | PubChem | |
URL | https://pubchem.ncbi.nlm.nih.gov | |
Description | Data deposited in or computed by PubChem | |
CAS No. |
4399-47-7 | |
Record name | Bromocyclobutane | |
Source | CAS Common Chemistry | |
URL | https://commonchemistry.cas.org/detail?cas_rn=4399-47-7 | |
Description | CAS Common Chemistry is an open community resource for accessing chemical information. Nearly 500,000 chemical substances from CAS REGISTRY cover areas of community interest, including common and frequently regulated chemicals, and those relevant to high school and undergraduate chemistry classes. This chemical information, curated by our expert scientists, is provided in alignment with our mission as a division of the American Chemical Society. | |
Explanation | The data from CAS Common Chemistry is provided under a CC-BY-NC 4.0 license, unless otherwise stated. | |
Record name | Bromocyclobutane | |
Source | ChemIDplus | |
URL | https://pubchem.ncbi.nlm.nih.gov/substance/?source=chemidplus&sourceid=0004399477 | |
Description | ChemIDplus is a free, web search system that provides access to the structure and nomenclature authority files used for the identification of chemical substances cited in National Library of Medicine (NLM) databases, including the TOXNET system. | |
Record name | Bromocyclobutane | |
Source | EPA DSSTox | |
URL | https://comptox.epa.gov/dashboard/DTXSID20196010 | |
Description | DSSTox provides a high quality public chemistry resource for supporting improved predictive toxicology. | |
Record name | Bromocyclobutane | |
Source | European Chemicals Agency (ECHA) | |
URL | https://echa.europa.eu/substance-information/-/substanceinfo/100.022.302 | |
Description | The European Chemicals Agency (ECHA) is an agency of the European Union which is the driving force among regulatory authorities in implementing the EU's groundbreaking chemicals legislation for the benefit of human health and the environment as well as for innovation and competitiveness. | |
Explanation | Use of the information, documents and data from the ECHA website is subject to the terms and conditions of this Legal Notice, and subject to other binding limitations provided for under applicable law, the information, documents and data made available on the ECHA website may be reproduced, distributed and/or used, totally or in part, for non-commercial purposes provided that ECHA is acknowledged as the source: "Source: European Chemicals Agency, http://echa.europa.eu/". Such acknowledgement must be included in each copy of the material. ECHA permits and encourages organisations and individuals to create links to the ECHA website under the following cumulative conditions: Links can only be made to webpages that provide a link to the Legal Notice page. | |
Retrosynthesis Analysis
AI-Powered Synthesis Planning: Our tool employs the Template_relevance Pistachio, Template_relevance Bkms_metabolic, Template_relevance Pistachio_ringbreaker, Template_relevance Reaxys, Template_relevance Reaxys_biocatalysis model, leveraging a vast database of chemical reactions to predict feasible synthetic routes.
One-Step Synthesis Focus: Specifically designed for one-step synthesis, it provides concise and direct routes for your target compounds, streamlining the synthesis process.
Accurate Predictions: Utilizing the extensive PISTACHIO, BKMS_METABOLIC, PISTACHIO_RINGBREAKER, REAXYS, REAXYS_BIOCATALYSIS database, our tool offers high-accuracy predictions, reflecting the latest in chemical research and data.
Strategy Settings
Precursor scoring | Relevance Heuristic |
---|---|
Min. plausibility | 0.01 |
Model | Template_relevance |
Template Set | Pistachio/Bkms_metabolic/Pistachio_ringbreaker/Reaxys/Reaxys_biocatalysis |
Top-N result to add to graph | 6 |
Feasible Synthetic Routes
Disclaimer and Information on In-Vitro Research Products
Please be aware that all articles and product information presented on BenchChem are intended solely for informational purposes. The products available for purchase on BenchChem are specifically designed for in-vitro studies, which are conducted outside of living organisms. In-vitro studies, derived from the Latin term "in glass," involve experiments performed in controlled laboratory settings using cells or tissues. It is important to note that these products are not categorized as medicines or drugs, and they have not received approval from the FDA for the prevention, treatment, or cure of any medical condition, ailment, or disease. We must emphasize that any form of bodily introduction of these products into humans or animals is strictly prohibited by law. It is essential to adhere to these guidelines to ensure compliance with legal and ethical standards in research and experimentation.