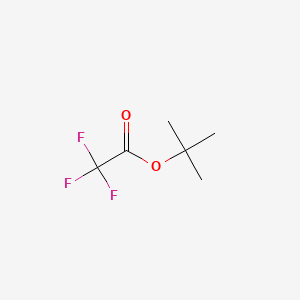
t-Butyl trifluoroacetate
Overview
Description
Mechanism of Action
- Role : The alkylation process introduces alkyl groups to the aromatic compounds, leading to changes in their chemical properties and reactivity .
- Process : The reagents are mixed at room temperature, and the solvent is evaporated after a few hours. This straightforward process allows for efficient alkylation .
- Downstream Effects : Alkylated aromatic compounds may exhibit altered chemical behavior, affecting subsequent reactions and biological processes .
Target of Action
Mode of Action
Biochemical Pathways
Result of Action
Biochemical Analysis
Biochemical Properties
t-Butyl trifluoroacetate plays a significant role in biochemical reactions, particularly in the protection of amine groups during peptide synthesis. It interacts with enzymes and proteins by forming stable carbamate linkages, which can be removed under specific conditions. This interaction is crucial for the synthesis of peptides and proteins, as it allows for the selective protection and deprotection of functional groups without affecting other parts of the molecule .
Cellular Effects
This compound can influence various cellular processes, including cell signaling pathways, gene expression, and cellular metabolism. It has been observed to inhibit the proliferation of osteoblasts and chondrocytes, indicating its potential impact on cell growth and differentiation . Additionally, its presence in cell culture systems can affect the activity of purified proteins, leading to changes in cellular functions.
Molecular Mechanism
The molecular mechanism of this compound involves its ability to form stable carbamate linkages with amine groups in proteins and peptides. This interaction can lead to the inhibition or activation of enzymes, depending on the specific context. For example, the removal of this compound protecting groups can be achieved using strong acids like trifluoroacetic acid, which cleaves the carbamate linkage and releases the free amine . This process is essential for the controlled synthesis and modification of peptides and proteins.
Temporal Effects in Laboratory Settings
In laboratory settings, the effects of this compound can change over time due to its stability and degradation properties. It is known to be stable under standard storage conditions but can degrade when exposed to strong acids or bases. The long-term effects of this compound on cellular function have been observed in in vitro studies, where its presence can lead to the gradual inhibition of cell proliferation and changes in gene expression .
Dosage Effects in Animal Models
The effects of this compound vary with different dosages in animal models. At low doses, it may have minimal impact on cellular functions, while higher doses can lead to toxic effects, including liver hypertrophy and other adverse reactions . These dosage-dependent effects highlight the importance of careful dosage control in experimental settings to avoid potential toxicity.
Metabolic Pathways
This compound is involved in various metabolic pathways, particularly those related to the synthesis and degradation of peptides and proteins. It interacts with enzymes such as proteases and peptidases, which can cleave the carbamate linkage and release the free amine. This interaction is crucial for the controlled modification of peptides and proteins in biochemical research .
Transport and Distribution
Within cells and tissues, this compound is transported and distributed through passive diffusion and active transport mechanisms. It can interact with transporters and binding proteins, which facilitate its movement across cell membranes and its localization within specific cellular compartments . This distribution is essential for its effective use in biochemical applications.
Subcellular Localization
This compound is localized within various subcellular compartments, including the cytoplasm and organelles such as the endoplasmic reticulum and Golgi apparatus. Its activity and function can be influenced by post-translational modifications and targeting signals that direct it to specific locations within the cell . This subcellular localization is critical for its role in the selective protection and deprotection of amine groups during peptide synthesis.
Preparation Methods
Synthetic Routes and Reaction Conditions: t-Butyl trifluoroacetate can be synthesized through the esterification of trifluoroacetic acid with tert-butyl alcohol. The reaction typically involves the use of a strong acid catalyst, such as sulfuric acid, to facilitate the esterification process. The reaction is carried out under reflux conditions to ensure complete conversion of the reactants to the desired ester .
Industrial Production Methods: In an industrial setting, the production of this compound may involve continuous flow processes to enhance efficiency and yield. Flow microreactor systems have been developed to introduce the tert-butoxycarbonyl group into various organic compounds, providing a more efficient and versatile method for large-scale production .
Chemical Reactions Analysis
Types of Reactions: t-Butyl trifluoroacetate undergoes several types of chemical reactions, including:
Alkylation: It acts as an efficient alkylating agent for aromatic compounds in the presence of trifluoroacetic acid.
Dealkylation: In the presence of strong acids, this compound can undergo dealkylation to form trifluoroacetic acid and tert-butyl cation.
Common Reagents and Conditions:
Dealkylation: Strong acids such as trifluoroacetic acid are used to facilitate the dealkylation process.
Major Products Formed:
Alkylation: The major products are alkylated aromatic compounds.
Dealkylation: The major products are trifluoroacetic acid and tert-butyl cation.
Scientific Research Applications
t-Butyl trifluoroacetate has a wide range of applications in scientific research, including:
Organic Synthesis: It is used as a reagent for introducing the trifluoroacetyl group into various substrates, which is valuable in the synthesis of pharmaceuticals and agrochemicals.
Peptide Synthesis: It serves as a protecting group for amines in peptide synthesis, allowing for selective deprotection under mild conditions.
Material Science: It is used in the preparation of fluorinated materials with unique properties, such as increased thermal stability and chemical resistance.
Comparison with Similar Compounds
Trifluoroacetic Acid: A strong acid with similar reactivity but lacks the tert-butyl group.
tert-Butyl Acetate: Similar structure but without the trifluoromethyl group, leading to different reactivity and applications.
Uniqueness: t-Butyl trifluoroacetate is unique due to the presence of both the tert-butyl and trifluoromethyl groups, which impart distinct chemical properties. The trifluoromethyl group enhances the compound’s reactivity and stability, while the tert-butyl group provides steric hindrance, making it a valuable reagent in organic synthesis .
Properties
IUPAC Name |
tert-butyl 2,2,2-trifluoroacetate | |
---|---|---|
Source | PubChem | |
URL | https://pubchem.ncbi.nlm.nih.gov | |
Description | Data deposited in or computed by PubChem | |
InChI |
InChI=1S/C6H9F3O2/c1-5(2,3)11-4(10)6(7,8)9/h1-3H3 | |
Source | PubChem | |
URL | https://pubchem.ncbi.nlm.nih.gov | |
Description | Data deposited in or computed by PubChem | |
InChI Key |
UQJLSMYQBOJUGG-UHFFFAOYSA-N | |
Source | PubChem | |
URL | https://pubchem.ncbi.nlm.nih.gov | |
Description | Data deposited in or computed by PubChem | |
Canonical SMILES |
CC(C)(C)OC(=O)C(F)(F)F | |
Source | PubChem | |
URL | https://pubchem.ncbi.nlm.nih.gov | |
Description | Data deposited in or computed by PubChem | |
Molecular Formula |
C6H9F3O2 | |
Source | PubChem | |
URL | https://pubchem.ncbi.nlm.nih.gov | |
Description | Data deposited in or computed by PubChem | |
DSSTOX Substance ID |
DTXSID40193061 | |
Record name | Acetic acid, trifluoro-, 1,1-dimethylethyl ester | |
Source | EPA DSSTox | |
URL | https://comptox.epa.gov/dashboard/DTXSID40193061 | |
Description | DSSTox provides a high quality public chemistry resource for supporting improved predictive toxicology. | |
Molecular Weight |
170.13 g/mol | |
Source | PubChem | |
URL | https://pubchem.ncbi.nlm.nih.gov | |
Description | Data deposited in or computed by PubChem | |
CAS No. |
400-52-2 | |
Record name | Acetic acid, trifluoro-, 1,1-dimethylethyl ester | |
Source | ChemIDplus | |
URL | https://pubchem.ncbi.nlm.nih.gov/substance/?source=chemidplus&sourceid=0000400522 | |
Description | ChemIDplus is a free, web search system that provides access to the structure and nomenclature authority files used for the identification of chemical substances cited in National Library of Medicine (NLM) databases, including the TOXNET system. | |
Record name | Acetic acid, trifluoro-, 1,1-dimethylethyl ester | |
Source | EPA DSSTox | |
URL | https://comptox.epa.gov/dashboard/DTXSID40193061 | |
Description | DSSTox provides a high quality public chemistry resource for supporting improved predictive toxicology. | |
Record name | tert-Butyl Trifluoroacetate | |
Source | European Chemicals Agency (ECHA) | |
URL | https://echa.europa.eu/information-on-chemicals | |
Description | The European Chemicals Agency (ECHA) is an agency of the European Union which is the driving force among regulatory authorities in implementing the EU's groundbreaking chemicals legislation for the benefit of human health and the environment as well as for innovation and competitiveness. | |
Explanation | Use of the information, documents and data from the ECHA website is subject to the terms and conditions of this Legal Notice, and subject to other binding limitations provided for under applicable law, the information, documents and data made available on the ECHA website may be reproduced, distributed and/or used, totally or in part, for non-commercial purposes provided that ECHA is acknowledged as the source: "Source: European Chemicals Agency, http://echa.europa.eu/". Such acknowledgement must be included in each copy of the material. ECHA permits and encourages organisations and individuals to create links to the ECHA website under the following cumulative conditions: Links can only be made to webpages that provide a link to the Legal Notice page. | |
Retrosynthesis Analysis
AI-Powered Synthesis Planning: Our tool employs the Template_relevance Pistachio, Template_relevance Bkms_metabolic, Template_relevance Pistachio_ringbreaker, Template_relevance Reaxys, Template_relevance Reaxys_biocatalysis model, leveraging a vast database of chemical reactions to predict feasible synthetic routes.
One-Step Synthesis Focus: Specifically designed for one-step synthesis, it provides concise and direct routes for your target compounds, streamlining the synthesis process.
Accurate Predictions: Utilizing the extensive PISTACHIO, BKMS_METABOLIC, PISTACHIO_RINGBREAKER, REAXYS, REAXYS_BIOCATALYSIS database, our tool offers high-accuracy predictions, reflecting the latest in chemical research and data.
Strategy Settings
Precursor scoring | Relevance Heuristic |
---|---|
Min. plausibility | 0.01 |
Model | Template_relevance |
Template Set | Pistachio/Bkms_metabolic/Pistachio_ringbreaker/Reaxys/Reaxys_biocatalysis |
Top-N result to add to graph | 6 |
Feasible Synthetic Routes
Q1: What makes t-butyl trifluoroacetate a useful reagent in organic synthesis?
A1: this compound acts as an efficient alkylating agent for aromatic compounds, especially in the presence of trifluoroacetic acid. [] This reaction proceeds readily at room temperature and results in high yields. [] This makes it a convenient reagent for introducing t-butyl groups into aromatic systems.
Q2: Are there any side reactions or challenges associated with using this compound in peptide synthesis?
A2: Yes, during the removal of t-butyl protecting groups from amino acids using trifluoroacetic acid, this compound is generated as a byproduct. [] This byproduct can unfortunately alkylate certain amino acids like methionine and tryptophan, potentially affecting the desired peptide sequence. []
Q3: Beyond alkylation, does this compound engage in other reactions relevant to organic chemistry?
A4: Interestingly, this compound participates in an equilibrium reaction with isobutene in the presence of trifluoroacetic acid. [] This equilibrium favors the formation of this compound at room temperature, implying that isobutene gas is unlikely to escape during deprotection reactions conducted in trifluoroacetic acid. []
Q4: What insights do the thermal decomposition studies of this compound offer?
A5: Research indicates that this compound decomposes thermally, primarily yielding isobutene and trifluoroacetic acid via a standard ester decomposition pathway. [] Importantly, the presence of the trifluoromethyl (CF3) group at the gamma carbon of the ester increases the transition state polarity, enhancing its reactivity and lowering the decomposition temperature compared to non-fluorinated analogs. []
Disclaimer and Information on In-Vitro Research Products
Please be aware that all articles and product information presented on BenchChem are intended solely for informational purposes. The products available for purchase on BenchChem are specifically designed for in-vitro studies, which are conducted outside of living organisms. In-vitro studies, derived from the Latin term "in glass," involve experiments performed in controlled laboratory settings using cells or tissues. It is important to note that these products are not categorized as medicines or drugs, and they have not received approval from the FDA for the prevention, treatment, or cure of any medical condition, ailment, or disease. We must emphasize that any form of bodily introduction of these products into humans or animals is strictly prohibited by law. It is essential to adhere to these guidelines to ensure compliance with legal and ethical standards in research and experimentation.