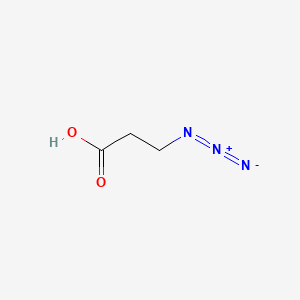
3-Azidopropanoic acid
Overview
Description
3-Azidopropanoic acid is an azido-containing building block . It contains an azide moiety and a carboxylic acid group . The azide group can undergo copper-catalyzed Click Chemistry reactions with alkyne groups . The terminal carboxylic acid group can react with primary amine groups in the presence of activators (e.g. EDC, or DCC), forming a stable amide bond .
Molecular Structure Analysis
The molecular formula of 3-Azidopropanoic acid is C3H5N3O2 . It has an average mass of 115.091 Da and a monoisotopic mass of 115.038177 Da .Chemical Reactions Analysis
The azide group in 3-Azidopropanoic acid can undergo copper-catalyzed Click Chemistry reactions with alkyne groups . The terminal carboxylic acid group can react with primary amine groups in the presence of activators such as EDC or HATU to form stable amide bonds .Physical And Chemical Properties Analysis
The physical and chemical properties of 3-Azidopropanoic acid include a density, boiling point, vapour pressure, enthalpy of vaporization, flash point, index of refraction, and molar refractivity . It has 5 H bond acceptors, 1 H bond donor, 3 freely rotating bonds, and 0 Rule of 5 violations . Its ACD/LogP is 0.00, ACD/LogD (pH 5.5) is -0.99, ACD/BCF (pH 5.5) is 1.00, ACD/KOC (pH 5.5) is 1.51, ACD/LogD (pH 7.4) is -2.74, ACD/BCF (pH 7.4) is 1.00, and ACD/KOC (pH 7.4) is 1.00 . Its polar surface area is 50 Å 2 .Scientific Research Applications
Click Chemistry
3-Azidopropanoic acid is an azido-containing building block that can be reacted with compounds containing alkyne groups through a copper (I)-catalyzed cycloaddition reaction, also known as click chemistry . This reaction is highly selective and efficient, making it a powerful tool in the field of synthetic chemistry.
Bioconjugation Strategies
The terminal azide group of 3-Azidopropanoic acid allows it to be used in various bioconjugation strategies . Bioconjugation is the process of chemically linking two biomolecules together, and it is a critical step in many biological research and drug development processes.
Synthesis of Chemical Probes
3-Azidopropanoic acid is useful for the synthesis of chemical probes . Chemical probes are small molecules that can bind to specific biological targets, allowing researchers to study the function and behavior of these targets in a biological system.
Crosslinking
3-Azidopropanoic acid can be used for crosslinking . Crosslinking is a process where polymers are chemically linked together, improving the properties of the material such as its strength and stability.
Formation of Stable Amide Bonds
3-Azidopropanoic acid can react with primary amine groups in the presence of activators (e.g. EDC, or DCC), forming a stable amide bond . This reaction is commonly used in peptide synthesis and other areas of organic chemistry.
Safety and Hazards
When handling 3-Azidopropanoic acid, it is advised to avoid dust formation, breathing mist, gas or vapours, and contacting with skin and eye . Use personal protective equipment, wear chemical impermeable gloves, ensure adequate ventilation, remove all sources of ignition, evacuate personnel to safe areas, and keep people away from and upwind of spill/leak .
Mechanism of Action
Target of Action
3-Azidopropanoic acid is a versatile building block in the field of bioconjugation . Its primary targets are compounds containing alkyne groups . The terminal azide group of 3-Azidopropanoic acid can react with these alkyne groups, forming a stable triazole ring .
Mode of Action
The interaction of 3-Azidopropanoic acid with its targets involves a copper (I)-catalyzed cycloaddition reaction, also known as click chemistry . This reaction allows the azide group of 3-Azidopropanoic acid to conjugate with alkyne groups, forming a stable triazole ring . Additionally, the terminal carboxylic acid group of 3-Azidopropanoic acid can react with primary amine groups in the presence of activators such as EDC or HATU, forming stable amide bonds .
Biochemical Pathways
The biochemical pathways affected by 3-Azidopropanoic acid are primarily related to the formation of stable triazole rings and amide bonds . These reactions can lead to the synthesis of new compounds and the modification of existing ones, enabling a wide range of bioconjugation strategies .
Pharmacokinetics
Its solubility in various solvents such as dmf, dmso, thf, chloroform, and dichloromethane suggests that it may have good bioavailability.
Result of Action
The result of 3-Azidopropanoic acid’s action is the formation of stable triazole rings and amide bonds . This enables the synthesis of new compounds and the modification of existing ones, which can be used in various applications such as crosslinking, synthesis of chemical probes, and other bioconjugation strategies .
Action Environment
The action of 3-Azidopropanoic acid can be influenced by various environmental factors. For instance, the presence of copper (I) is necessary for the cycloaddition reaction . Additionally, the presence of activators such as EDC or HATU is required for the formation of amide bonds
properties
IUPAC Name |
3-azidopropanoic acid | |
---|---|---|
Source | PubChem | |
URL | https://pubchem.ncbi.nlm.nih.gov | |
Description | Data deposited in or computed by PubChem | |
InChI |
InChI=1S/C3H5N3O2/c4-6-5-2-1-3(7)8/h1-2H2,(H,7,8) | |
Source | PubChem | |
URL | https://pubchem.ncbi.nlm.nih.gov | |
Description | Data deposited in or computed by PubChem | |
InChI Key |
YIPVUXAMZQBALD-UHFFFAOYSA-N | |
Source | PubChem | |
URL | https://pubchem.ncbi.nlm.nih.gov | |
Description | Data deposited in or computed by PubChem | |
Canonical SMILES |
C(CN=[N+]=[N-])C(=O)O | |
Source | PubChem | |
URL | https://pubchem.ncbi.nlm.nih.gov | |
Description | Data deposited in or computed by PubChem | |
Molecular Formula |
C3H5N3O2 | |
Source | PubChem | |
URL | https://pubchem.ncbi.nlm.nih.gov | |
Description | Data deposited in or computed by PubChem | |
DSSTOX Substance ID |
DTXSID50171759 | |
Record name | Azidopropionic acid | |
Source | EPA DSSTox | |
URL | https://comptox.epa.gov/dashboard/DTXSID50171759 | |
Description | DSSTox provides a high quality public chemistry resource for supporting improved predictive toxicology. | |
Molecular Weight |
115.09 g/mol | |
Source | PubChem | |
URL | https://pubchem.ncbi.nlm.nih.gov | |
Description | Data deposited in or computed by PubChem | |
Product Name |
3-Azidopropanoic acid | |
CAS RN |
18523-47-2 | |
Record name | 3-Azidopropanoic acid | |
Source | CAS Common Chemistry | |
URL | https://commonchemistry.cas.org/detail?cas_rn=18523-47-2 | |
Description | CAS Common Chemistry is an open community resource for accessing chemical information. Nearly 500,000 chemical substances from CAS REGISTRY cover areas of community interest, including common and frequently regulated chemicals, and those relevant to high school and undergraduate chemistry classes. This chemical information, curated by our expert scientists, is provided in alignment with our mission as a division of the American Chemical Society. | |
Explanation | The data from CAS Common Chemistry is provided under a CC-BY-NC 4.0 license, unless otherwise stated. | |
Record name | Azidopropionic acid | |
Source | ChemIDplus | |
URL | https://pubchem.ncbi.nlm.nih.gov/substance/?source=chemidplus&sourceid=0018523472 | |
Description | ChemIDplus is a free, web search system that provides access to the structure and nomenclature authority files used for the identification of chemical substances cited in National Library of Medicine (NLM) databases, including the TOXNET system. | |
Record name | Azidopropionic acid | |
Source | EPA DSSTox | |
URL | https://comptox.epa.gov/dashboard/DTXSID50171759 | |
Description | DSSTox provides a high quality public chemistry resource for supporting improved predictive toxicology. | |
Record name | 3-AZIDOPROPANOIC ACID | |
Source | European Chemicals Agency (ECHA) | |
URL | https://echa.europa.eu/information-on-chemicals | |
Description | The European Chemicals Agency (ECHA) is an agency of the European Union which is the driving force among regulatory authorities in implementing the EU's groundbreaking chemicals legislation for the benefit of human health and the environment as well as for innovation and competitiveness. | |
Explanation | Use of the information, documents and data from the ECHA website is subject to the terms and conditions of this Legal Notice, and subject to other binding limitations provided for under applicable law, the information, documents and data made available on the ECHA website may be reproduced, distributed and/or used, totally or in part, for non-commercial purposes provided that ECHA is acknowledged as the source: "Source: European Chemicals Agency, http://echa.europa.eu/". Such acknowledgement must be included in each copy of the material. ECHA permits and encourages organisations and individuals to create links to the ECHA website under the following cumulative conditions: Links can only be made to webpages that provide a link to the Legal Notice page. | |
Record name | AZIDOPROPIONIC ACID | |
Source | FDA Global Substance Registration System (GSRS) | |
URL | https://gsrs.ncats.nih.gov/ginas/app/beta/substances/PXM5HY6VUN | |
Description | The FDA Global Substance Registration System (GSRS) enables the efficient and accurate exchange of information on what substances are in regulated products. Instead of relying on names, which vary across regulatory domains, countries, and regions, the GSRS knowledge base makes it possible for substances to be defined by standardized, scientific descriptions. | |
Explanation | Unless otherwise noted, the contents of the FDA website (www.fda.gov), both text and graphics, are not copyrighted. They are in the public domain and may be republished, reprinted and otherwise used freely by anyone without the need to obtain permission from FDA. Credit to the U.S. Food and Drug Administration as the source is appreciated but not required. | |
Retrosynthesis Analysis
AI-Powered Synthesis Planning: Our tool employs the Template_relevance Pistachio, Template_relevance Bkms_metabolic, Template_relevance Pistachio_ringbreaker, Template_relevance Reaxys, Template_relevance Reaxys_biocatalysis model, leveraging a vast database of chemical reactions to predict feasible synthetic routes.
One-Step Synthesis Focus: Specifically designed for one-step synthesis, it provides concise and direct routes for your target compounds, streamlining the synthesis process.
Accurate Predictions: Utilizing the extensive PISTACHIO, BKMS_METABOLIC, PISTACHIO_RINGBREAKER, REAXYS, REAXYS_BIOCATALYSIS database, our tool offers high-accuracy predictions, reflecting the latest in chemical research and data.
Strategy Settings
Precursor scoring | Relevance Heuristic |
---|---|
Min. plausibility | 0.01 |
Model | Template_relevance |
Template Set | Pistachio/Bkms_metabolic/Pistachio_ringbreaker/Reaxys/Reaxys_biocatalysis |
Top-N result to add to graph | 6 |
Feasible Synthetic Routes
Q & A
Q1: How does 3-azidopropanoic acid interact with carbodiimides, and what are the downstream effects?
A1: 3-azidopropanoic acid reacts with carbodiimides, such as DIC (N,N'-Diisopropylcarbodiimide), through a unique mechanism. Instead of the expected amide formation, the reaction leads to the formation of a six-membered ring intermediate. [, ] This intermediate acts as a versatile precursor for synthesizing various aza-heterocycles, including mono-, bi-, and tricyclic compounds. [, ] The azide group in 3-azidopropanoic acid acts as a "pseudo leaving group" in this process.
Q2: What makes this reaction pathway with 3-azidopropanoic acid significant in organic synthesis?
A2: This reaction pathway is significant because it offers a one-step method for synthesizing diverse aza-heterocycles. [, ] Traditional methods often involve multiple steps and harsh reaction conditions. This new approach, utilizing the unique reactivity of 3-azidopropanoic acid with carbodiimides, provides a more efficient and potentially milder route to these important chemical structures.
Disclaimer and Information on In-Vitro Research Products
Please be aware that all articles and product information presented on BenchChem are intended solely for informational purposes. The products available for purchase on BenchChem are specifically designed for in-vitro studies, which are conducted outside of living organisms. In-vitro studies, derived from the Latin term "in glass," involve experiments performed in controlled laboratory settings using cells or tissues. It is important to note that these products are not categorized as medicines or drugs, and they have not received approval from the FDA for the prevention, treatment, or cure of any medical condition, ailment, or disease. We must emphasize that any form of bodily introduction of these products into humans or animals is strictly prohibited by law. It is essential to adhere to these guidelines to ensure compliance with legal and ethical standards in research and experimentation.