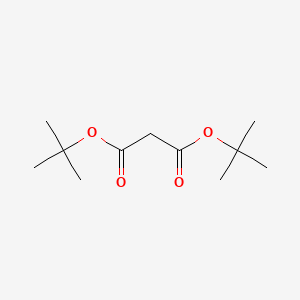
Di-tert-butyl malonate
Overview
Description
Di-tert-Butyl Malonate is a ptential porogen for porogen-derived membranes.
Scientific Research Applications
Synthesis and Chemical Reactions
Di-tert-butyl malonate is utilized in various chemical syntheses and reactions. It serves as an intermediate in the formation of several chemical compounds, including esterification products like tert-butyl acetate and di-tert-butyl succinate, among others. Its involvement in the esterification of dicarboxylic acids is particularly noted (McCloskey et al., 2003).
Pharmaceutical Applications
In pharmaceutical research, this compound is used in the synthesis of Boc-protected amines through a process known as the Curtius rearrangement. This process is important for creating protected amino acids, showcasing the compound's role in developing pharmaceutical intermediates (Lebel & Leogane, 2005).
Material Science and Photolithography
In the field of material science and photolithography, this compound has been utilized in the development of photodefinable polymers. It plays a crucial role in the synthesis of novel alkyl malonated copolymers used in deep-UV photoresists, which are essential in the fabrication of microelectronic devices (Choi et al., 1996).
Environmental Chemistry
The compound is also studied in environmental chemistry, particularly in the context of its photo-oxidation in the presence and absence of nitrogen dioxide. This research provides insights into the behavior of this compound in atmospheric conditions and its interaction with other environmental pollutants (Henao et al., 2016).
Organic Synthesis
In organic synthesis, this compound is used as a versatile carboxylating reagent. Its effectiveness in trapping carbon nucleophiles and producing a variety of tert-butyl carboxylates highlights its significance in the synthesis of complex organic molecules (Augustine et al., 2009).
Mechanism of Action
Target of Action
Di-tert-butyl malonate is a chemical compound with the formula C11H20O4 . It is used as a pharmaceutical intermediate . .
Mode of Action
It is known to be used in the total synthesis of (+/−)-actinophyllic acid . It also serves as a precursor for metal-organic chemical vapor deposition of HfO2 and ZrO2 thin films .
Biochemical Pathways
It is involved in the synthesis of actinophyllic acid
Pharmacokinetics
Its physical and chemical properties such as its molecular weight (21627 g/mol) , boiling point (110-111 °C/22 mmHg) , and density (0.966 g/mL at 25 °C) can influence its bioavailability.
Result of Action
It is known to be used in the synthesis of actinophyllic acid , which suggests it may have a role in the formation of complex organic compounds.
Action Environment
It is known to be a combustible liquid , suggesting that it may be sensitive to heat and open flames. Its efficacy and stability could potentially be influenced by environmental conditions such as temperature and exposure to light.
Biochemical Analysis
Biochemical Properties
Di-tert-butyl malonate plays a significant role in biochemical reactions, particularly as a precursor in the synthesis of other compounds. It interacts with various enzymes, proteins, and biomolecules. For instance, this compound is used in the total synthesis of actinophyllic acid and as a precursor for metal-organic chemical vapor deposition of HfO2 and ZrO2 thin films . The interactions of this compound with these biomolecules are primarily based on its ability to form esters and other derivatives, which can participate in various biochemical pathways.
Cellular Effects
This compound influences various cellular processes, including cell signaling pathways, gene expression, and cellular metabolism. It has been observed to protect against cardiac ischemia-reperfusion injury by enhancing intracellular delivery and inhibiting mitochondrial reactive oxygen species production . This indicates that this compound can modulate cellular functions and protect cells from oxidative stress.
Molecular Mechanism
The molecular mechanism of this compound involves its interaction with biomolecules at the molecular level. It acts as a prodrug that is hydrolyzed to release malonate, which inhibits succinate dehydrogenase (SDH) and reduces mitochondrial reactive oxygen species production . This inhibition of SDH prevents the accumulation of succinate and subsequent oxidative stress, thereby protecting cells from ischemia-reperfusion injury.
Temporal Effects in Laboratory Settings
In laboratory settings, the effects of this compound change over time. The compound is relatively stable, but its hydrolysis to malonate can influence its long-term effects on cellular function. Studies have shown that this compound can provide sustained protection against oxidative stress when administered at the point of reperfusion . This indicates that the temporal effects of this compound are closely related to its stability and hydrolysis rate.
Dosage Effects in Animal Models
The effects of this compound vary with different dosages in animal models. At lower doses, it can effectively inhibit succinate dehydrogenase and protect against oxidative stress. At higher doses, this compound may exhibit toxic or adverse effects . It is essential to determine the optimal dosage to achieve the desired protective effects without causing toxicity.
Metabolic Pathways
This compound is involved in metabolic pathways related to the inhibition of succinate dehydrogenase. It interacts with enzymes and cofactors involved in the tricarboxylic acid (TCA) cycle, particularly succinate dehydrogenase . By inhibiting this enzyme, this compound can modulate metabolic flux and reduce the production of reactive oxygen species.
Transport and Distribution
Within cells and tissues, this compound is transported and distributed through various mechanisms. It can be taken up by cells and hydrolyzed to release malonate, which then exerts its effects on mitochondrial function . The distribution of this compound within tissues is influenced by its ability to cross cell membranes and reach target sites.
Subcellular Localization
This compound is localized within specific subcellular compartments, particularly mitochondria. Its hydrolysis to malonate allows it to target mitochondrial succinate dehydrogenase and inhibit its activity . This subcellular localization is crucial for its protective effects against oxidative stress and ischemia-reperfusion injury.
Properties
IUPAC Name |
ditert-butyl propanedioate | |
---|---|---|
Source | PubChem | |
URL | https://pubchem.ncbi.nlm.nih.gov | |
Description | Data deposited in or computed by PubChem | |
InChI |
InChI=1S/C11H20O4/c1-10(2,3)14-8(12)7-9(13)15-11(4,5)6/h7H2,1-6H3 | |
Source | PubChem | |
URL | https://pubchem.ncbi.nlm.nih.gov | |
Description | Data deposited in or computed by PubChem | |
InChI Key |
CLPHAYNBNTVRDI-UHFFFAOYSA-N | |
Source | PubChem | |
URL | https://pubchem.ncbi.nlm.nih.gov | |
Description | Data deposited in or computed by PubChem | |
Canonical SMILES |
CC(C)(C)OC(=O)CC(=O)OC(C)(C)C | |
Source | PubChem | |
URL | https://pubchem.ncbi.nlm.nih.gov | |
Description | Data deposited in or computed by PubChem | |
Molecular Formula |
C11H20O4 | |
Source | PubChem | |
URL | https://pubchem.ncbi.nlm.nih.gov | |
Description | Data deposited in or computed by PubChem | |
DSSTOX Substance ID |
DTXSID8060244 | |
Record name | Propanedioic acid, bis(1,1-dimethylethyl) ester | |
Source | EPA DSSTox | |
URL | https://comptox.epa.gov/dashboard/DTXSID8060244 | |
Description | DSSTox provides a high quality public chemistry resource for supporting improved predictive toxicology. | |
Molecular Weight |
216.27 g/mol | |
Source | PubChem | |
URL | https://pubchem.ncbi.nlm.nih.gov | |
Description | Data deposited in or computed by PubChem | |
CAS No. |
541-16-2 | |
Record name | 1,3-Bis(1,1-dimethylethyl) propanedioate | |
Source | CAS Common Chemistry | |
URL | https://commonchemistry.cas.org/detail?cas_rn=541-16-2 | |
Description | CAS Common Chemistry is an open community resource for accessing chemical information. Nearly 500,000 chemical substances from CAS REGISTRY cover areas of community interest, including common and frequently regulated chemicals, and those relevant to high school and undergraduate chemistry classes. This chemical information, curated by our expert scientists, is provided in alignment with our mission as a division of the American Chemical Society. | |
Explanation | The data from CAS Common Chemistry is provided under a CC-BY-NC 4.0 license, unless otherwise stated. | |
Record name | Di-tert-butyl malonate | |
Source | ChemIDplus | |
URL | https://pubchem.ncbi.nlm.nih.gov/substance/?source=chemidplus&sourceid=0000541162 | |
Description | ChemIDplus is a free, web search system that provides access to the structure and nomenclature authority files used for the identification of chemical substances cited in National Library of Medicine (NLM) databases, including the TOXNET system. | |
Record name | Propanedioic acid, 1,3-bis(1,1-dimethylethyl) ester | |
Source | EPA Chemicals under the TSCA | |
URL | https://www.epa.gov/chemicals-under-tsca | |
Description | EPA Chemicals under the Toxic Substances Control Act (TSCA) collection contains information on chemicals and their regulations under TSCA, including non-confidential content from the TSCA Chemical Substance Inventory and Chemical Data Reporting. | |
Record name | Propanedioic acid, bis(1,1-dimethylethyl) ester | |
Source | EPA DSSTox | |
URL | https://comptox.epa.gov/dashboard/DTXSID8060244 | |
Description | DSSTox provides a high quality public chemistry resource for supporting improved predictive toxicology. | |
Record name | Bis(1,1-dimethylethyl) malonate | |
Source | European Chemicals Agency (ECHA) | |
URL | https://echa.europa.eu/substance-information/-/substanceinfo/100.007.973 | |
Description | The European Chemicals Agency (ECHA) is an agency of the European Union which is the driving force among regulatory authorities in implementing the EU's groundbreaking chemicals legislation for the benefit of human health and the environment as well as for innovation and competitiveness. | |
Explanation | Use of the information, documents and data from the ECHA website is subject to the terms and conditions of this Legal Notice, and subject to other binding limitations provided for under applicable law, the information, documents and data made available on the ECHA website may be reproduced, distributed and/or used, totally or in part, for non-commercial purposes provided that ECHA is acknowledged as the source: "Source: European Chemicals Agency, http://echa.europa.eu/". Such acknowledgement must be included in each copy of the material. ECHA permits and encourages organisations and individuals to create links to the ECHA website under the following cumulative conditions: Links can only be made to webpages that provide a link to the Legal Notice page. | |
Record name | DI-TERT-BUTYL MALONATE | |
Source | FDA Global Substance Registration System (GSRS) | |
URL | https://gsrs.ncats.nih.gov/ginas/app/beta/substances/7E9XWT9380 | |
Description | The FDA Global Substance Registration System (GSRS) enables the efficient and accurate exchange of information on what substances are in regulated products. Instead of relying on names, which vary across regulatory domains, countries, and regions, the GSRS knowledge base makes it possible for substances to be defined by standardized, scientific descriptions. | |
Explanation | Unless otherwise noted, the contents of the FDA website (www.fda.gov), both text and graphics, are not copyrighted. They are in the public domain and may be republished, reprinted and otherwise used freely by anyone without the need to obtain permission from FDA. Credit to the U.S. Food and Drug Administration as the source is appreciated but not required. | |
Retrosynthesis Analysis
AI-Powered Synthesis Planning: Our tool employs the Template_relevance Pistachio, Template_relevance Bkms_metabolic, Template_relevance Pistachio_ringbreaker, Template_relevance Reaxys, Template_relevance Reaxys_biocatalysis model, leveraging a vast database of chemical reactions to predict feasible synthetic routes.
One-Step Synthesis Focus: Specifically designed for one-step synthesis, it provides concise and direct routes for your target compounds, streamlining the synthesis process.
Accurate Predictions: Utilizing the extensive PISTACHIO, BKMS_METABOLIC, PISTACHIO_RINGBREAKER, REAXYS, REAXYS_BIOCATALYSIS database, our tool offers high-accuracy predictions, reflecting the latest in chemical research and data.
Strategy Settings
Precursor scoring | Relevance Heuristic |
---|---|
Min. plausibility | 0.01 |
Model | Template_relevance |
Template Set | Pistachio/Bkms_metabolic/Pistachio_ringbreaker/Reaxys/Reaxys_biocatalysis |
Top-N result to add to graph | 6 |
Feasible Synthetic Routes
Disclaimer and Information on In-Vitro Research Products
Please be aware that all articles and product information presented on BenchChem are intended solely for informational purposes. The products available for purchase on BenchChem are specifically designed for in-vitro studies, which are conducted outside of living organisms. In-vitro studies, derived from the Latin term "in glass," involve experiments performed in controlled laboratory settings using cells or tissues. It is important to note that these products are not categorized as medicines or drugs, and they have not received approval from the FDA for the prevention, treatment, or cure of any medical condition, ailment, or disease. We must emphasize that any form of bodily introduction of these products into humans or animals is strictly prohibited by law. It is essential to adhere to these guidelines to ensure compliance with legal and ethical standards in research and experimentation.