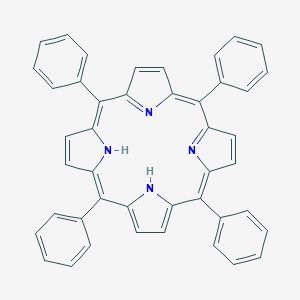
Tetraphenylporphyrin
Overview
Description
Tetraphenylporphyrin is a meso-substituted porphyrin and a phenylporphyrin.
Mechanism of Action
Target of Action
Tetraphenylporphyrin (TPP) is a synthetic heterocyclic compound that resembles naturally occurring porphyrins . Porphyrins are dyes and cofactors found in hemoglobin and cytochromes and are related to chlorophyll and vitamin B12 . TPP’s primary targets are various types of bacteria, where it acts as a photosensitizer for photodynamic therapy .
Mode of Action
TPP interacts with its targets through a process known as photodynamic inactivation (PDI) . In this process, TPP, when exposed to light, generates reactive oxygen species (ROS) that can inactivate bacteria . The introduction of substituents of different natures makes it possible to vary the physicochemical properties of porphyrins in a wide range .
Biochemical Pathways
The biochemical pathways affected by TPP involve the generation of ROS. These ROS can cause damage to cellular components, including proteins, lipids, and nucleic acids, leading to cell death . Furthermore, TPP can participate in the formation of S1-macroheterocycle-S2 bridging structures and effectively participate in virus inactivation .
Pharmacokinetics
The pharmacokinetics of TPP, particularly its absorption, distribution, metabolism, and excretion (ADME) properties, are influenced by its formulation. For instance, when TPP is loaded into poly(lactic-co-glycolic acid) nanoparticles, it exhibits a biphasic release profile and a release mechanism via Fick diffusion . This formulation also enhances TPP’s bioavailability and allows for its specific accumulation in tissues .
Result of Action
The result of TPP’s action is the inactivation of bacteria. It has been shown to have significant antitumor activity and the ability to generate ROS . Moreover, TPP-loaded nanoparticles have been found to exhibit high safety in in vitro hemolysis assays .
Action Environment
The action of TPP is influenced by various environmental factors. For instance, the nature of the solvent, concentration, and pH value can affect the chemical activity of TPP . Additionally, the physicochemical properties of TPP and the pathophysiological properties of the tumor microenvironment influence the drug toxicity and pharmacokinetics .
Biochemical Analysis
Biochemical Properties
Tetraphenylporphyrin has unique photophysical and biochemical properties . It can interact with various biomolecules, including amino acids, leading to compounds that can participate in the formation of S1-macroheterocycle-S2 bridging structures and effectively participate in virus inactivation . The base properties of asymmetrically substituted tetraphenylporphyrins increase due to the electronic effect of protonated forms of substituents at the macrocycle periphery .
Cellular Effects
The cellular effects of this compound are largely due to its ability to generate high levels of reactive oxygen with a low dark toxicity . These properties make it a robust photosensitizing agent, which can be used in photodynamic therapy for cancer treatment .
Molecular Mechanism
This compound exerts its effects at the molecular level through various mechanisms. For instance, it can engage in binding interactions with biomolecules, leading to enzyme inhibition or activation . It can also induce changes in gene expression .
Temporal Effects in Laboratory Settings
The effects of this compound can change over time in laboratory settings. For example, the nature of the solvent, concentration, and pH value can affect the chemical activity of porphyrin . Amino acid fragments can also affect the protolytic equilibrium of porphyrins in acidic and basic media .
Dosage Effects in Animal Models
The effects of this compound can vary with different dosages in animal models
Transport and Distribution
This compound can be transported and distributed within cells and tissues
Subcellular Localization
This compound can be localized in various subcellular compartments . For instance, with exclusive localization in mitochondria, it can induce rapid mitochondrial membrane potential transition (MPT), leading to cellular apoptosis .
Biological Activity
Tetraphenylporphyrin (TPP) is a synthetic porphyrin that has garnered significant interest in the field of biochemistry due to its versatile biological activities. This article explores the various biological activities associated with TPP, including its antimicrobial properties, interactions with proteins, and potential therapeutic applications.
1. Overview of this compound
This compound is a member of the porphyrin family, characterized by its four phenyl groups attached to a porphyrin core. Its structure allows for modifications that enhance its solubility and biological compatibility. TPP and its derivatives have been extensively studied for their potential applications in photodynamic therapy (PDT), antimicrobial treatments, and as probes in biochemical research.
2. Antimicrobial Activity
TPP exhibits notable antimicrobial properties, particularly when activated through photodynamic mechanisms. Studies have demonstrated its effectiveness against various bacterial strains, including multidrug-resistant pathogens.
2.1 Photodynamic Antimicrobial Effects
A recent study evaluated the photodynamic antimicrobial activity of a TPP derivative against Pseudomonas aeruginosa and Staphylococcus aureus. The results indicated that the minimum inhibitory concentration (MIC) significantly decreased under light irradiation conditions:
Bacterial Strain | MIC (µg/mL) without Irradiation | MIC (µg/mL) with Irradiation |
---|---|---|
S. aureus | 109.30 | 69.42 |
P. aeruginosa | 402.90 | 54.71 |
The study concluded that TPP effectively damages bacterial cell structures through singlet oxygen generation when activated by light, leading to increased bacterial mortality .
2.2 Interaction with Proteins
Research has also shown that TPP can form complexes with proteins, enhancing its antimicrobial efficacy. A study involving the haemoprotein HasA demonstrated that TPP derivatives could inhibit the growth of Pseudomonas aeruginosa by sequestering iron, which is essential for bacterial proliferation:
TPP Derivative | Inhibition of PAO1 Growth |
---|---|
CoTPP-HasA | High |
GaTPP-HasA | Moderate |
These findings suggest that TPP's ability to interact with biological macromolecules may open new avenues for developing antimicrobial agents against resistant strains .
3. Antitumor and Antioxidant Properties
Apart from its antimicrobial activity, TPP has been investigated for its potential antitumor effects. Studies indicate that certain TPP derivatives can induce apoptosis in cancer cells while exhibiting low toxicity towards normal cells.
The mechanisms by which TPP exerts its antitumor effects include:
- Generation of Reactive Oxygen Species (ROS) : Upon light activation, TPP generates ROS that can induce oxidative stress in cancer cells.
- Inhibition of Cellular Proliferation : TPP has been shown to interfere with cell cycle progression in various cancer cell lines.
4. Case Studies and Research Findings
Several case studies highlight the diverse applications of TPP in biological systems:
- Case Study 1 : A study assessed the impact of axially ligated zirconium(IV) this compound on bacterial strains, revealing significant antibacterial activity against Sclerotium rolfsii and other pathogens .
- Case Study 2 : In vitro studies demonstrated that TPP derivatives could effectively bind to cytochrome c, showcasing their potential as biochemical probes for studying protein interactions .
5. Conclusion
This compound represents a promising compound with multifaceted biological activities, particularly in antimicrobial and antitumor applications. Its ability to generate reactive oxygen species upon light activation positions it as a valuable candidate for photodynamic therapy and other therapeutic interventions. Ongoing research is likely to uncover further applications and enhance our understanding of this versatile compound's role in biological systems.
Properties
IUPAC Name |
5,10,15,20-tetraphenyl-21,23-dihydroporphyrin | |
---|---|---|
Source | PubChem | |
URL | https://pubchem.ncbi.nlm.nih.gov | |
Description | Data deposited in or computed by PubChem | |
InChI |
InChI=1S/C44H30N4/c1-5-13-29(14-6-1)41-33-21-23-35(45-33)42(30-15-7-2-8-16-30)37-25-27-39(47-37)44(32-19-11-4-12-20-32)40-28-26-38(48-40)43(31-17-9-3-10-18-31)36-24-22-34(41)46-36/h1-28,45,48H | |
Source | PubChem | |
URL | https://pubchem.ncbi.nlm.nih.gov | |
Description | Data deposited in or computed by PubChem | |
InChI Key |
YNHJECZULSZAQK-UHFFFAOYSA-N | |
Source | PubChem | |
URL | https://pubchem.ncbi.nlm.nih.gov | |
Description | Data deposited in or computed by PubChem | |
Canonical SMILES |
C1=CC=C(C=C1)C2=C3C=CC(=C(C4=NC(=C(C5=CC=C(N5)C(=C6C=CC2=N6)C7=CC=CC=C7)C8=CC=CC=C8)C=C4)C9=CC=CC=C9)N3 | |
Source | PubChem | |
URL | https://pubchem.ncbi.nlm.nih.gov | |
Description | Data deposited in or computed by PubChem | |
Molecular Formula |
C44H30N4 | |
Source | PubChem | |
URL | https://pubchem.ncbi.nlm.nih.gov | |
Description | Data deposited in or computed by PubChem | |
DSSTOX Substance ID |
DTXSID2061272 | |
Record name | 21H,23H-Porphine, 5,10,15,20-tetraphenyl- | |
Source | EPA DSSTox | |
URL | https://comptox.epa.gov/dashboard/DTXSID2061272 | |
Description | DSSTox provides a high quality public chemistry resource for supporting improved predictive toxicology. | |
Molecular Weight |
614.7 g/mol | |
Source | PubChem | |
URL | https://pubchem.ncbi.nlm.nih.gov | |
Description | Data deposited in or computed by PubChem | |
Physical Description |
Purple crystalline powder; [Alfa Aesar MSDS] | |
Record name | Tetraphenylporphine | |
Source | Haz-Map, Information on Hazardous Chemicals and Occupational Diseases | |
URL | https://haz-map.com/Agents/17952 | |
Description | Haz-Map® is an occupational health database designed for health and safety professionals and for consumers seeking information about the adverse effects of workplace exposures to chemical and biological agents. | |
Explanation | Copyright (c) 2022 Haz-Map(R). All rights reserved. Unless otherwise indicated, all materials from Haz-Map are copyrighted by Haz-Map(R). No part of these materials, either text or image may be used for any purpose other than for personal use. Therefore, reproduction, modification, storage in a retrieval system or retransmission, in any form or by any means, electronic, mechanical or otherwise, for reasons other than personal use, is strictly prohibited without prior written permission. | |
CAS No. |
917-23-7 | |
Record name | Tetraphenylporphyrin | |
Source | ChemIDplus | |
URL | https://pubchem.ncbi.nlm.nih.gov/substance/?source=chemidplus&sourceid=0000917237 | |
Description | ChemIDplus is a free, web search system that provides access to the structure and nomenclature authority files used for the identification of chemical substances cited in National Library of Medicine (NLM) databases, including the TOXNET system. | |
Record name | Tetraphenylporphyrin | |
Source | DTP/NCI | |
URL | https://dtp.cancer.gov/dtpstandard/servlet/dwindex?searchtype=NSC&outputformat=html&searchlist=18506 | |
Description | The NCI Development Therapeutics Program (DTP) provides services and resources to the academic and private-sector research communities worldwide to facilitate the discovery and development of new cancer therapeutic agents. | |
Explanation | Unless otherwise indicated, all text within NCI products is free of copyright and may be reused without our permission. Credit the National Cancer Institute as the source. | |
Record name | 21H,23H-Porphine, 5,10,15,20-tetraphenyl- | |
Source | EPA Chemicals under the TSCA | |
URL | https://www.epa.gov/chemicals-under-tsca | |
Description | EPA Chemicals under the Toxic Substances Control Act (TSCA) collection contains information on chemicals and their regulations under TSCA, including non-confidential content from the TSCA Chemical Substance Inventory and Chemical Data Reporting. | |
Record name | 21H,23H-Porphine, 5,10,15,20-tetraphenyl- | |
Source | EPA DSSTox | |
URL | https://comptox.epa.gov/dashboard/DTXSID2061272 | |
Description | DSSTox provides a high quality public chemistry resource for supporting improved predictive toxicology. | |
Record name | 5,10,15,20-tetraphenyl-21H,23H-porphine | |
Source | European Chemicals Agency (ECHA) | |
URL | https://echa.europa.eu/substance-information/-/substanceinfo/100.011.842 | |
Description | The European Chemicals Agency (ECHA) is an agency of the European Union which is the driving force among regulatory authorities in implementing the EU's groundbreaking chemicals legislation for the benefit of human health and the environment as well as for innovation and competitiveness. | |
Explanation | Use of the information, documents and data from the ECHA website is subject to the terms and conditions of this Legal Notice, and subject to other binding limitations provided for under applicable law, the information, documents and data made available on the ECHA website may be reproduced, distributed and/or used, totally or in part, for non-commercial purposes provided that ECHA is acknowledged as the source: "Source: European Chemicals Agency, http://echa.europa.eu/". Such acknowledgement must be included in each copy of the material. ECHA permits and encourages organisations and individuals to create links to the ECHA website under the following cumulative conditions: Links can only be made to webpages that provide a link to the Legal Notice page. | |
Record name | Tetraphenylporphyrin | |
Source | FDA Global Substance Registration System (GSRS) | |
URL | https://gsrs.ncats.nih.gov/ginas/app/beta/substances/SDB2SH8G5K | |
Description | The FDA Global Substance Registration System (GSRS) enables the efficient and accurate exchange of information on what substances are in regulated products. Instead of relying on names, which vary across regulatory domains, countries, and regions, the GSRS knowledge base makes it possible for substances to be defined by standardized, scientific descriptions. | |
Explanation | Unless otherwise noted, the contents of the FDA website (www.fda.gov), both text and graphics, are not copyrighted. They are in the public domain and may be republished, reprinted and otherwise used freely by anyone without the need to obtain permission from FDA. Credit to the U.S. Food and Drug Administration as the source is appreciated but not required. | |
Synthesis routes and methods
Procedure details
Retrosynthesis Analysis
AI-Powered Synthesis Planning: Our tool employs the Template_relevance Pistachio, Template_relevance Bkms_metabolic, Template_relevance Pistachio_ringbreaker, Template_relevance Reaxys, Template_relevance Reaxys_biocatalysis model, leveraging a vast database of chemical reactions to predict feasible synthetic routes.
One-Step Synthesis Focus: Specifically designed for one-step synthesis, it provides concise and direct routes for your target compounds, streamlining the synthesis process.
Accurate Predictions: Utilizing the extensive PISTACHIO, BKMS_METABOLIC, PISTACHIO_RINGBREAKER, REAXYS, REAXYS_BIOCATALYSIS database, our tool offers high-accuracy predictions, reflecting the latest in chemical research and data.
Strategy Settings
Precursor scoring | Relevance Heuristic |
---|---|
Min. plausibility | 0.01 |
Model | Template_relevance |
Template Set | Pistachio/Bkms_metabolic/Pistachio_ringbreaker/Reaxys/Reaxys_biocatalysis |
Top-N result to add to graph | 6 |
Feasible Synthetic Routes
ANone: The molecular formula of tetraphenylporphyrin is C44H30N4. Its molecular weight is 614.74 g/mol.
A: Tetraphenylporphyrins are characterized by intense absorption bands in the visible region of the electromagnetic spectrum, known as Soret and Q bands. These bands arise from electronic transitions within the porphyrin macrocycle. Additionally, they exhibit characteristic peaks in infrared (IR) and nuclear magnetic resonance (NMR) spectra, which can be used to identify and characterize the molecule. []
A: Substituents on the phenyl rings of tetraphenylporphyrins can influence the energy levels of the porphyrin's electronic states, leading to shifts in the absorption bands. Electron-donating groups generally cause a redshift (shift to longer wavelengths), while electron-withdrawing groups cause a blueshift (shift to shorter wavelengths). [, , , ] For example, tetrakis(2-naphthyl) porphyrin phosphorus(V) complex (NP) shows a redshift in the absorption band compared to dimethoxyP(V)this compound (PP) due to the presence of the naphthyl moiety. [] The extent of these shifts depends on the nature and position of the substituents. Furthermore, changes in the porphyrin's conformation, such as distortions from planarity, can also impact the absorption spectrum. [, ]
A: Nuclear magnetic resonance (NMR) studies indicate that this compound (H2TPP) interacts with fullerene C60 at both the pyrrole and phenyl hydrogen sites in solution. [] The strength and nature of these interactions are influenced by factors such as the solvent and the presence of substituents on the phenyl rings of the porphyrin. For instance, electron-donating groups, like methoxy (-OCH3), promote interaction at the pyrrole site. [, ]
A: The specific metal ion coordinated to the porphyrin ring plays a crucial role in the bimolecular organization of tetraphenylporphyrins on gold surfaces. [] Studies using electrochemical scanning tunneling microscopy (EC-STM) have revealed that while mixtures of CoTPP and CuTPP form compositionally disordered arrays, mixtures of CoTPP and ZnTPP exhibit site-selective adsorption, leading to distinct domains of each complex on the gold surface. [] This behavior suggests that the metal ion influences the interaction strength between the porphyrin molecules and the gold substrate.
A: Cobalt(III) tetraphenylporphyrins can catalyze hydride transfer reactions from hydride donors, such as tributyltin hydride, to substrates like 10-methylacridinium ion. The mechanism involves the formation of a hydridocobalt(III) this compound intermediate, which then transfers the hydride to the substrate. []
A: Yes, cobalt(III) tetraphenylporphyrins can catalyze the hydrometallation of alkenes and alkynes using tributyltin hydride. The reaction proceeds via the formation of a hydridocobalt(III) porphyrin intermediate, followed by hydrogen transfer to the unsaturated bond. This process leads to the formation of organocobalt(III) porphyrins with high regioselectivity, influenced by the stability of the radical intermediates formed during the hydrogen transfer step. []
A: Titanate nanotubes (TiNTs) sensitized with different metalloporphyrins exhibit varying photocatalytic activities. [] For instance, in the photodegradation of methyl orange, TiNTs sensitized with zinc this compound (ZnTPP) displayed the highest activity, followed by free-base TPP, NiTPP, and MnTPP. These differences in activity arise from the varying abilities of the central metal ions in the porphyrin ring to absorb visible light and facilitate photoelectron transfer processes. []
A: The size of the central metal ion can influence the nonplanar distortion of OATPPs. [] Larger metal ions tend to reduce the nonplanarity of the porphyrin macrocycle. This effect is attributed to the interplay between the steric interactions of the peripheral substituents and the size of the central metal ion. Crystallographic studies and molecular mechanics calculations have confirmed this metal-dependent conformational flexibility in OATPPs. []
A: Nonplanar distortions in porphyrins can significantly alter their electronic structure, leading to changes in their optical spectra. These distortions disrupt the conjugation within the porphyrin macrocycle, affecting the energy levels of molecular orbitals involved in electronic transitions. Consequently, nonplanar porphyrins often exhibit redshifts in their absorption spectra compared to their planar counterparts. [, , ] Theoretical calculations, including molecular mechanics and molecular orbital methods, are valuable tools for predicting and understanding the relationship between porphyrin conformation and optical properties. [, ]
A: Yes, tetraphenylporphyrins can be incorporated into polymer matrices to create photoconductive materials. [] For example, copolymers containing this compound and carbazole moieties have been synthesized and used as charge-generating layers in photoreceptors. The presence of the porphyrin units enhances the photosensitivity of the polymer films due to their ability to absorb light and generate charge carriers. []
A: The incorporation of silver nanoparticles into poly(1-trimethylsilyl-1-propyne) nanofibers embedded with palladium(II) or platinum(II) meso-tetraphenylporphyrins enhances their oxygen-sensing capabilities. [] The silver nanoparticles improve the phosphorescence quantum yields and sensitivity of the porphyrin molecules toward oxygen, leading to faster response times, higher Stern–Volmer constants (KSV), and lower limits of detection. []
A: Tetraphenylporphyrins can undergo various reactions at the periphery, including electrophilic aromatic substitutions, oxidations, and reductions. For instance, they can be halogenated, nitrated, or sulfonated at the phenyl rings. [, , , ] The reactivity of the porphyrin ring can be modulated by the central metal ion and the nature of the substituents on the phenyl rings.
A: The oxidation state of the metal center in a this compound complex can be altered using appropriate oxidizing or reducing agents. For example, treatment of an N-fused this compound rhenium(I) tricarbonyl complex with trimethylamine N-oxide leads to the oxidation of the rhenium center from +1 to +7, forming a stable rhenium(VII) trioxo complex. [] Similarly, iron(III) porphyrin π-cation radicals can be generated by reacting iron(III) porphyrins with oxidizing agents like bromine. []
A: The synthesis of tetraphenylporphyrins often involves multi-step procedures and can be challenging due to the formation of various isomers and byproducts. Achieving high yields and purity requires careful control of reaction conditions, including temperature, solvent, and catalyst. [] Additionally, the purification of porphyrins can be challenging due to their tendency to aggregate.
Disclaimer and Information on In-Vitro Research Products
Please be aware that all articles and product information presented on BenchChem are intended solely for informational purposes. The products available for purchase on BenchChem are specifically designed for in-vitro studies, which are conducted outside of living organisms. In-vitro studies, derived from the Latin term "in glass," involve experiments performed in controlled laboratory settings using cells or tissues. It is important to note that these products are not categorized as medicines or drugs, and they have not received approval from the FDA for the prevention, treatment, or cure of any medical condition, ailment, or disease. We must emphasize that any form of bodily introduction of these products into humans or animals is strictly prohibited by law. It is essential to adhere to these guidelines to ensure compliance with legal and ethical standards in research and experimentation.