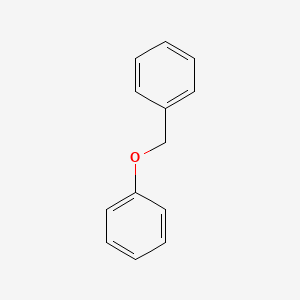
Benzyl phenyl ether
Overview
Description
Benzyl phenyl ether is a reactive organic oxygenate, containing an ether as a functional group . It is present in subbituminous and bituminous coals . It reacts with aluminium bromide in chlorobenzene solution to afford a mixture of phenol, o-benzyl phenol, and dichlorodiphenylmethane .
Synthesis Analysis
Ethers are usually prepared from alcohols or their conjugate bases . One important procedure, known as the Williamson Ether Synthesis, proceeds by an S N 2 reaction of an alkoxide nucleophile with an alkyl halide . In the case of Benzyl phenyl ether, it reacts with aluminium bromide in chlorobenzene solution .Molecular Structure Analysis
The molecular structure of Benzyl phenyl ether includes the arrangement of atoms and the chemical bonds that hold the atoms together . The molecule contains a total of 27 bonds. There are 15 non-H bonds, 12 multiple bonds, 3 rotatable bonds, 12 aromatic bonds, 2 six-membered rings, and 1 aromatic ether .Chemical Reactions Analysis
Benzyl phenyl ether reacts with aluminium bromide in chlorobenzene solution to afford a mixture of phenol, o-benzyl phenol, and dichlorodiphenylmethane . It is a useful model compound in catalytic chemistry to represent the a-O4 ether bond in lignin and coal .Physical And Chemical Properties Analysis
Benzyl phenyl ether has a boiling point of 286-287 °C (lit.) and a melting point of 39-41 °C (lit.) . Its molecular weight is 184.23 g/mol .Scientific Research Applications
Lignin Model Compound
As a model compound, BPE represents the α-O-4 ether bond in lignin and coal . This is crucial for understanding the behavior of these materials during chemical processing. BPE’s weak ether bond, which is one of the most thermo-labile compounds in lignin and low-rank coal, is essential for studying the thermal stability and decomposition pathways of lignin.
Catalytic Chemistry
In catalytic chemistry, BPE is used to study the influence of alkali carbonates on reaction pathways in biomass conversion . These insights are vital for optimizing the conversion processes to enhance the yield of desired products.
Decomposition to Aromatics
The decomposition of BPE to aromatics has been investigated using cesium-exchanged heteropolyacid catalysts . This research is part of efforts to find efficient catalysts that can break down complex organic molecules into simpler aromatic compounds, which are valuable in various industrial applications.
Photo-Claisen Rearrangement Studies
BPE undergoes photo-Claisen rearrangement, which has been studied in cation-exchanged Y zeolites and polyethylenes of differing crystallinities . These studies help in understanding the photoreactivity of organic compounds and can lead to the development of new photochemical processes.
Reaction with Aluminum Bromide
When reacted with aluminum bromide in chlorobenzene solution, BPE affords a mixture of phenol, o-benzyl phenol, and dichlorodiphenylmethane . This reaction is significant for the synthesis of these compounds, which have various industrial and research applications.
Mechanism of Action
Target of Action
Benzyl phenyl ether is a reactive organic oxygenate, containing an ether as a functional group . It is present in subbituminous and bituminous coals . The primary targets of benzyl phenyl ether are aluminium bromide in chlorobenzene solution .
Mode of Action
Benzyl phenyl ether interacts with its targets through a reaction process. It reacts with aluminium bromide in chlorobenzene solution to afford a mixture of phenol, o-benzyl phenol, and dichlorodiphenylmethane . This reaction represents the a-O4 ether bond in lignin and coal .
Biochemical Pathways
The hydrogenolysis of benzyl phenyl ether (BPE), a model lignin ether, closely follows that of solution-based reactions . The primary products of this reaction are toluene, phenol, and cyclohexanol . This reaction pathway is crucial for the mild depolymerization of lignin using reductive mechanocatalysis .
Pharmacokinetics
The hydrogenolysis rate of benzyl phenyl ether has been shown to be largely insensitive to the final nickel particle size . This suggests that the compound’s Absorption, Distribution, Metabolism, and Excretion (ADME) properties may be influenced by factors other than particle size.
Result of Action
The result of the action of benzyl phenyl ether is the production of phenol, o-benzyl phenol, and dichlorodiphenylmethane . These products are formed due to the interaction of benzyl phenyl ether with aluminium bromide in chlorobenzene solution .
Action Environment
The action of benzyl phenyl ether can be influenced by environmental factors. For instance, the influence of alkali carbonates, common additives in biomass conversion, on the reaction pathways of BPE in superheated water has been reported .
Safety and Hazards
Future Directions
properties
IUPAC Name |
phenoxymethylbenzene | |
---|---|---|
Source | PubChem | |
URL | https://pubchem.ncbi.nlm.nih.gov | |
Description | Data deposited in or computed by PubChem | |
InChI |
InChI=1S/C13H12O/c1-3-7-12(8-4-1)11-14-13-9-5-2-6-10-13/h1-10H,11H2 | |
Source | PubChem | |
URL | https://pubchem.ncbi.nlm.nih.gov | |
Description | Data deposited in or computed by PubChem | |
InChI Key |
BOTNYLSAWDQNEX-UHFFFAOYSA-N | |
Source | PubChem | |
URL | https://pubchem.ncbi.nlm.nih.gov | |
Description | Data deposited in or computed by PubChem | |
Canonical SMILES |
C1=CC=C(C=C1)COC2=CC=CC=C2 | |
Source | PubChem | |
URL | https://pubchem.ncbi.nlm.nih.gov | |
Description | Data deposited in or computed by PubChem | |
Molecular Formula |
C13H12O | |
Source | PubChem | |
URL | https://pubchem.ncbi.nlm.nih.gov | |
Description | Data deposited in or computed by PubChem | |
DSSTOX Substance ID |
DTXSID70870813 | |
Record name | Benzyloxybenzene | |
Source | EPA DSSTox | |
URL | https://comptox.epa.gov/dashboard/DTXSID70870813 | |
Description | DSSTox provides a high quality public chemistry resource for supporting improved predictive toxicology. | |
Molecular Weight |
184.23 g/mol | |
Source | PubChem | |
URL | https://pubchem.ncbi.nlm.nih.gov | |
Description | Data deposited in or computed by PubChem | |
Product Name |
Benzyl phenyl ether | |
CAS RN |
946-80-5, 31324-44-4 | |
Record name | Benzyl phenyl ether | |
Source | ChemIDplus | |
URL | https://pubchem.ncbi.nlm.nih.gov/substance/?source=chemidplus&sourceid=0000946805 | |
Description | ChemIDplus is a free, web search system that provides access to the structure and nomenclature authority files used for the identification of chemical substances cited in National Library of Medicine (NLM) databases, including the TOXNET system. | |
Record name | Phenoxytoluene | |
Source | ChemIDplus | |
URL | https://pubchem.ncbi.nlm.nih.gov/substance/?source=chemidplus&sourceid=0031324444 | |
Description | ChemIDplus is a free, web search system that provides access to the structure and nomenclature authority files used for the identification of chemical substances cited in National Library of Medicine (NLM) databases, including the TOXNET system. | |
Record name | Benzyl phenyl ether | |
Source | DTP/NCI | |
URL | https://dtp.cancer.gov/dtpstandard/servlet/dwindex?searchtype=NSC&outputformat=html&searchlist=77971 | |
Description | The NCI Development Therapeutics Program (DTP) provides services and resources to the academic and private-sector research communities worldwide to facilitate the discovery and development of new cancer therapeutic agents. | |
Explanation | Unless otherwise indicated, all text within NCI products is free of copyright and may be reused without our permission. Credit the National Cancer Institute as the source. | |
Record name | Benzyloxybenzene | |
Source | EPA DSSTox | |
URL | https://comptox.epa.gov/dashboard/DTXSID70870813 | |
Description | DSSTox provides a high quality public chemistry resource for supporting improved predictive toxicology. | |
Record name | Benzyl Phenyl Ether | |
Source | European Chemicals Agency (ECHA) | |
URL | https://echa.europa.eu/information-on-chemicals | |
Description | The European Chemicals Agency (ECHA) is an agency of the European Union which is the driving force among regulatory authorities in implementing the EU's groundbreaking chemicals legislation for the benefit of human health and the environment as well as for innovation and competitiveness. | |
Explanation | Use of the information, documents and data from the ECHA website is subject to the terms and conditions of this Legal Notice, and subject to other binding limitations provided for under applicable law, the information, documents and data made available on the ECHA website may be reproduced, distributed and/or used, totally or in part, for non-commercial purposes provided that ECHA is acknowledged as the source: "Source: European Chemicals Agency, http://echa.europa.eu/". Such acknowledgement must be included in each copy of the material. ECHA permits and encourages organisations and individuals to create links to the ECHA website under the following cumulative conditions: Links can only be made to webpages that provide a link to the Legal Notice page. | |
Record name | BENZYL PHENYL ETHER | |
Source | FDA Global Substance Registration System (GSRS) | |
URL | https://gsrs.ncats.nih.gov/ginas/app/beta/substances/BUE863N0L8 | |
Description | The FDA Global Substance Registration System (GSRS) enables the efficient and accurate exchange of information on what substances are in regulated products. Instead of relying on names, which vary across regulatory domains, countries, and regions, the GSRS knowledge base makes it possible for substances to be defined by standardized, scientific descriptions. | |
Explanation | Unless otherwise noted, the contents of the FDA website (www.fda.gov), both text and graphics, are not copyrighted. They are in the public domain and may be republished, reprinted and otherwise used freely by anyone without the need to obtain permission from FDA. Credit to the U.S. Food and Drug Administration as the source is appreciated but not required. | |
Synthesis routes and methods I
Procedure details
Synthesis routes and methods II
Procedure details
Synthesis routes and methods III
Procedure details
Synthesis routes and methods IV
Procedure details
Synthesis routes and methods V
Procedure details
Retrosynthesis Analysis
AI-Powered Synthesis Planning: Our tool employs the Template_relevance Pistachio, Template_relevance Bkms_metabolic, Template_relevance Pistachio_ringbreaker, Template_relevance Reaxys, Template_relevance Reaxys_biocatalysis model, leveraging a vast database of chemical reactions to predict feasible synthetic routes.
One-Step Synthesis Focus: Specifically designed for one-step synthesis, it provides concise and direct routes for your target compounds, streamlining the synthesis process.
Accurate Predictions: Utilizing the extensive PISTACHIO, BKMS_METABOLIC, PISTACHIO_RINGBREAKER, REAXYS, REAXYS_BIOCATALYSIS database, our tool offers high-accuracy predictions, reflecting the latest in chemical research and data.
Strategy Settings
Precursor scoring | Relevance Heuristic |
---|---|
Min. plausibility | 0.01 |
Model | Template_relevance |
Template Set | Pistachio/Bkms_metabolic/Pistachio_ringbreaker/Reaxys/Reaxys_biocatalysis |
Top-N result to add to graph | 6 |
Feasible Synthetic Routes
Q & A
ANone: Benzyl phenyl ether has a molecular formula of C13H12O and a molecular weight of 184.24 g/mol.
A: Yes, studies have used techniques like infrared spectroscopy [], 13C nuclear magnetic resonance (NMR) [], and magic angle spinning (MAS) NMR spectroscopy [] to characterize benzyl phenyl ether and monitor its reactions.
A: Benzyl phenyl ether undergoes different reaction pathways depending on the solvent. In subcritical and supercritical water, both pyrolysis and hydrolysis occur. In contrast, only pyrolysis occurs in supercritical methanol [].
A: Benzyl phenyl ether serves as a model for the α-O-4 linkages present in lignin, a major component of lignocellulosic biomass [, ]. Understanding its conversion can provide insights into depolymerizing lignin for biofuel and renewable chemical production.
A: Metal triflates, particularly hafnium triflate, catalyze the conversion of benzyl phenyl ether into larger phenolic compounds (C12-C22) through a one-step C-O breaking and C-C coupling process [].
ANone: The choice of catalyst significantly affects product selectivity:
- Copper-doped porous metal oxides (CuPMOs) in supercritical methanol catalyze lignin disassembly, but also promote aromatic ring hydrogenations and methylations [, , ].
- Adding samarium(III) to CuPMOs enhances aryl ether hydrogenolysis, particularly when incorporated within the catalyst matrix [].
- Introducing formic acid to the CuPMO system, while suppressing hydrogenolysis, improves selectivity towards aromatic products [].
- Nickel supported on nitrogen-doped porous carbon with cerium oxide (Ni–CeO2/CN) exhibits high activity and selectivity for cleaving C–O ether bonds, producing phenol and toluene from benzyl phenyl ether at low temperatures [].
A: A two-step hydrodeoxygenation process shows promise []:
A: Mechanocatalysis, specifically ball milling with supported nickel catalysts, enables solvent-free hydrogenolysis of benzyl phenyl ether []. This green chemistry approach holds potential for sustainable biomass valorization.
ANone: DFT calculations provide insights into:
- The mechanism of aryl ether hydrogenolysis []
- Explaining the higher activity of Pd/Ce-BTC compared to Pd/CeO2 in catalyzing benzyl phenyl ether conversion []
A: The presence and nature of substituents on the aromatic rings influence the reaction rate and selectivity. For instance, electron-withdrawing groups generally increase the activation energy barrier for C-O bond cleavage []. Studies have investigated the relationship between substituents and reaction rates in reactions with various reagents like N-chlorosuccinimide [] and 2,3-dichloro-5,6-dicyanoquinone (DDQ) [].
A: While the provided research doesn't delve into specific formulation strategies for benzyl phenyl ether, its limited solubility in water is a known challenge []. Employing co-solvent systems, such as ethanol/water mixtures, can enhance solubility and facilitate reactions like electrochemical degradation []. Further research might explore formulation approaches to improve its processability and applications in various fields.
ANone: Various methods have been employed to track the reaction progress and identify products:
- Gas chromatography (GC): Quantifies the products formed during thermolysis and helps determine conversion rates [, ].
- Gas chromatography-mass spectrometry (GC/MS): Identifies the products and intermediates formed during reactions [].
- Operando magic-angle spinning NMR spectroscopy: Allows for real-time monitoring of reaction progress and identification of intermediates at elevated temperatures and pressures [].
A: Benzyl phenyl ether exhibits poor solubility in water, hindering its use in aqueous-based reactions []. This necessitates the use of co-solvents or alternative reaction media to enhance its solubility and facilitate desired transformations.
A: Initial studies on benzyl phenyl ether primarily focused on its synthesis and fundamental reactivity [, ]. With increasing interest in biomass conversion, its role as a lignin model compound gained prominence [, , ]. Recent research explores novel catalytic approaches, including those utilizing mechanochemistry and electrochemistry [, ], to efficiently and selectively convert benzyl phenyl ether into valuable chemicals.
ANone: The knowledge gained from studying benzyl phenyl ether transcends disciplinary boundaries. For example:
Disclaimer and Information on In-Vitro Research Products
Please be aware that all articles and product information presented on BenchChem are intended solely for informational purposes. The products available for purchase on BenchChem are specifically designed for in-vitro studies, which are conducted outside of living organisms. In-vitro studies, derived from the Latin term "in glass," involve experiments performed in controlled laboratory settings using cells or tissues. It is important to note that these products are not categorized as medicines or drugs, and they have not received approval from the FDA for the prevention, treatment, or cure of any medical condition, ailment, or disease. We must emphasize that any form of bodily introduction of these products into humans or animals is strictly prohibited by law. It is essential to adhere to these guidelines to ensure compliance with legal and ethical standards in research and experimentation.