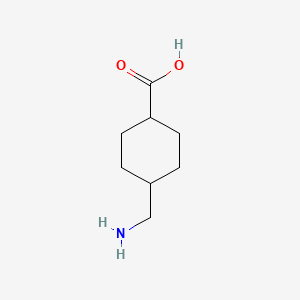
Tranexamic acid
Overview
Description
Tranexamic acid (TXA) is an antifibrinolytic agent that works by blocking the breakdown of blood clots, which prevents bleeding . It is used to treat heavy menstrual bleeding in women . TXA is a synthetic derivative of lysine that exerts antifibrinolytic effects by blocking lysine binding sites on plasminogen molecules, inhibiting the interaction of plasminogen with formed plasmin and fibrin .
Synthesis Analysis
A direct and efficient method for the preparation of key intermediate methyl 4- (acetamidomethyl)benzoate by one-pot hydrogenation and acylation in acetic anhydride using Ni/Al 2 O 3 as a catalyst has been developed .
Molecular Structure Analysis
Tranexamic acid has a simple chemical structure consisting of a cyclohexane ring with an amino group and a carboxylic acid group attached . Its chemical formula is C8H15NO2 . The molecular weight of tranexamic acid is 157.21 g/mol .
Chemical Reactions Analysis
Tranexamic acid is an antifibrinolytic that prevents or reduces bleeding by impairing fibrin dissolution . It is a synthetic derivative of lysine that exerts antifibrinolytic effects by blocking lysine binding sites on plasminogen molecules, inhibiting the interaction of plasminogen with formed plasmin and fibrin .
Physical And Chemical Properties Analysis
Tranexamic acid is typically found as a white or almost white crystalline powder . The melting point of tranexamic acid is approximately 300-305°C (572-581°F) . It is sparingly soluble in water and ethanol but more soluble in methanol .
Scientific Research Applications
Treatment of Melasma and Sun-Induced Skin Hyperpigmentation
Tranexamic acid has been shown to be effective against melasma, a skin condition that causes brown patches on the face. It is considered first-line pharmacotherapy for this condition . Topically applied TXA has also been found to be effective against skin hyperpigmentation caused by sunburn and inflammation . The skin-lightening mechanism involves the suppression of cytokine/chemical mediator production, which stimulates melanin production .
Use in Plastic Surgery
Tranexamic acid is a versatile antifibrinolytic agent that is widely used in modern surgeries . In plastic surgery, intravenous (IV) TXA administration results in less blood loss, reduced need for transfusion, and better surgical fields . It does not increase the risk of adverse events .
Prevention of Bleeding Complications
Tranexamic acid has demonstrated efficacy in preventing bleeding complications across various hemostatic challenges while minimizing adverse effects . It works by permanently inhibiting lysine binding sites on plasminogen molecules, which prohibits plasminogen activator (PA) from converting plasminogen to plasmin .
Military Application in Trauma Emergency
The use of TXA with blood component-based resuscitation following combat injury results in improved measures of coagulopathy and survival . This benefit is most prominent in patients requiring massive transfusion .
Mechanism of Action
Target of Action
Tranexamic acid (TXA) is a synthetic derivative of lysine . Its primary target is plasminogen , a protein that plays a crucial role in the fibrinolysis process . By binding to plasminogen, TXA prevents the conversion of plasminogen to its proteolytically activated form, plasmin .
Mode of Action
TXA acts as a lysine mimetic . It competitively inhibits the activation of plasminogen to plasmin . At higher concentrations, it behaves as a noncompetitive inhibitor of plasmin . TXA binds more strongly than aminocaproic acid (a similar antifibrinolytic) to both the strong and weak receptor sites of the plasminogen molecule .
Biochemical Pathways
The primary biochemical pathway affected by TXA is the fibrinolysis pathway . By inhibiting the conversion of plasminogen to plasmin, TXA prevents the degradation of fibrin, a protein that forms a mesh-like structure during blood clotting . This action helps to stabilize blood clots and reduce bleeding. In addition, TXA has been found to lower complement activation and decrease the need for C1 esterase inhibitors, thereby reducing inflammation associated with hereditary angioedema .
Pharmacokinetics
TXA exhibits a bioavailability of approximately 45% when taken orally . It reaches peak plasma concentrations in about 3 hours . The initial volume of distribution of TXA is 0.18 L/kg, and its steady-state volume of distribution is 0.39 L/kg . The elimination half-life of TXA ranges from 2 to 11 hours . Over 95% of TXA is excreted in the urine as unchanged drug .
Result of Action
The primary result of TXA’s action is the reduction or prevention of hemorrhagic episodes , especially in the context of hyperfibrinolytic disorders . It is used to treat conditions such as hereditary angioedema, heavy menstrual bleeding, and significant bleeding in trauma cases . TXA’s action helps to stabilize blood clots, reduce bleeding, and in some cases, reduce inflammation .
Action Environment
The efficacy and stability of TXA can be influenced by various environmental factors. For instance, the presence of other medications can impact TXA’s effectiveness. It’s important to consider shared decision-making in patients taking TXA and combined hormonal contraceptives . Furthermore, the timing of TXA administration can significantly impact its efficacy. Early treatment (within 1 hour of trauma) is associated with the greatest benefit, while treatment after 3 hours appears to increase the risk of death due to bleeding .
Safety and Hazards
Tranexamic acid causes skin irritation, serious eye irritation, and may cause respiratory irritation . Adverse effects include venous thromboembolic events, such as deep vein thrombosis and pulmonary embolism, stroke, myocardial infarction, renal failure or dysfunction, seizures, and mild gastrointestinal symptoms (nausea, vomiting, diarrhea) .
Future Directions
Tranexamic acid dosing regimens should be decreased in patients with chronic kidney dysfunction secondary to reduced clearance and drug accumulation . Optimal dosing for cardiac surgical patients has been recommended . Additional research is required to determine dosing regimens in major noncardiac surgery and plasma concentration levels associated with inducing seizures .
properties
IUPAC Name |
4-(aminomethyl)cyclohexane-1-carboxylic acid | |
---|---|---|
Source | PubChem | |
URL | https://pubchem.ncbi.nlm.nih.gov | |
Description | Data deposited in or computed by PubChem | |
InChI |
InChI=1S/C8H15NO2/c9-5-6-1-3-7(4-2-6)8(10)11/h6-7H,1-5,9H2,(H,10,11) | |
Source | PubChem | |
URL | https://pubchem.ncbi.nlm.nih.gov | |
Description | Data deposited in or computed by PubChem | |
InChI Key |
GYDJEQRTZSCIOI-UHFFFAOYSA-N | |
Source | PubChem | |
URL | https://pubchem.ncbi.nlm.nih.gov | |
Description | Data deposited in or computed by PubChem | |
Canonical SMILES |
C1CC(CCC1CN)C(=O)O | |
Source | PubChem | |
URL | https://pubchem.ncbi.nlm.nih.gov | |
Description | Data deposited in or computed by PubChem | |
Molecular Formula |
C8H15NO2 | |
Source | PubChem | |
URL | https://pubchem.ncbi.nlm.nih.gov | |
Description | Data deposited in or computed by PubChem | |
DSSTOX Substance ID |
DTXSID3045350, DTXSID50904827 | |
Record name | Tranexamic acid | |
Source | EPA DSSTox | |
URL | https://comptox.epa.gov/dashboard/DTXSID3045350 | |
Description | DSSTox provides a high quality public chemistry resource for supporting improved predictive toxicology. | |
Record name | 4-(Aminomethyl)-cyclohexanecarboxylic acid | |
Source | EPA DSSTox | |
URL | https://comptox.epa.gov/dashboard/DTXSID50904827 | |
Description | DSSTox provides a high quality public chemistry resource for supporting improved predictive toxicology. | |
Molecular Weight |
157.21 g/mol | |
Source | PubChem | |
URL | https://pubchem.ncbi.nlm.nih.gov | |
Description | Data deposited in or computed by PubChem | |
Mechanism of Action |
Tranexamic acid competitively and reversibly inhibits the activation of plasminogen via binding at several distinct sites, including four or five low-affinity sites and one high-affinity site, the latter of which is involved in its binding to fibrin. The binding of plasminogen to fibrin induces fibrinolysis - by occupying the necessary binding sites tranexamic acid prevents this dissolution of fibrin, thereby stabilizing the clot and preventing hemorrhage. | |
Record name | Tranexamic acid | |
Source | DrugBank | |
URL | https://www.drugbank.ca/drugs/DB00302 | |
Description | The DrugBank database is a unique bioinformatics and cheminformatics resource that combines detailed drug (i.e. chemical, pharmacological and pharmaceutical) data with comprehensive drug target (i.e. sequence, structure, and pathway) information. | |
Explanation | Creative Common's Attribution-NonCommercial 4.0 International License (http://creativecommons.org/licenses/by-nc/4.0/legalcode) | |
Product Name |
Tranexamic acid | |
CAS RN |
701-54-2, 1197-17-7, 1197-18-8 | |
Record name | 4-(Aminomethyl)cyclohexanecarboxylic acid | |
Source | CAS Common Chemistry | |
URL | https://commonchemistry.cas.org/detail?cas_rn=701-54-2 | |
Description | CAS Common Chemistry is an open community resource for accessing chemical information. Nearly 500,000 chemical substances from CAS REGISTRY cover areas of community interest, including common and frequently regulated chemicals, and those relevant to high school and undergraduate chemistry classes. This chemical information, curated by our expert scientists, is provided in alignment with our mission as a division of the American Chemical Society. | |
Explanation | The data from CAS Common Chemistry is provided under a CC-BY-NC 4.0 license, unless otherwise stated. | |
Record name | Cyclohexanecarboxylic acid, 4-(aminomethyl)- | |
Source | ChemIDplus | |
URL | https://pubchem.ncbi.nlm.nih.gov/substance/?source=chemidplus&sourceid=0000701542 | |
Description | ChemIDplus is a free, web search system that provides access to the structure and nomenclature authority files used for the identification of chemical substances cited in National Library of Medicine (NLM) databases, including the TOXNET system. | |
Record name | cis-Tranexamic acid | |
Source | ChemIDplus | |
URL | https://pubchem.ncbi.nlm.nih.gov/substance/?source=chemidplus&sourceid=0001197177 | |
Description | ChemIDplus is a free, web search system that provides access to the structure and nomenclature authority files used for the identification of chemical substances cited in National Library of Medicine (NLM) databases, including the TOXNET system. | |
Record name | Tranexamic acid [USAN:USP:INN:BAN:JAN] | |
Source | ChemIDplus | |
URL | https://pubchem.ncbi.nlm.nih.gov/substance/?source=chemidplus&sourceid=0001197188 | |
Description | ChemIDplus is a free, web search system that provides access to the structure and nomenclature authority files used for the identification of chemical substances cited in National Library of Medicine (NLM) databases, including the TOXNET system. | |
Record name | Tranexamic acid | |
Source | DrugBank | |
URL | https://www.drugbank.ca/drugs/DB00302 | |
Description | The DrugBank database is a unique bioinformatics and cheminformatics resource that combines detailed drug (i.e. chemical, pharmacological and pharmaceutical) data with comprehensive drug target (i.e. sequence, structure, and pathway) information. | |
Explanation | Creative Common's Attribution-NonCommercial 4.0 International License (http://creativecommons.org/licenses/by-nc/4.0/legalcode) | |
Record name | tranexamic acid | |
Source | DTP/NCI | |
URL | https://dtp.cancer.gov/dtpstandard/servlet/dwindex?searchtype=NSC&outputformat=html&searchlist=758176 | |
Description | The NCI Development Therapeutics Program (DTP) provides services and resources to the academic and private-sector research communities worldwide to facilitate the discovery and development of new cancer therapeutic agents. | |
Explanation | Unless otherwise indicated, all text within NCI products is free of copyright and may be reused without our permission. Credit the National Cancer Institute as the source. | |
Record name | tranexamic acid | |
Source | DTP/NCI | |
URL | https://dtp.cancer.gov/dtpstandard/servlet/dwindex?searchtype=NSC&outputformat=html&searchlist=291305 | |
Description | The NCI Development Therapeutics Program (DTP) provides services and resources to the academic and private-sector research communities worldwide to facilitate the discovery and development of new cancer therapeutic agents. | |
Explanation | Unless otherwise indicated, all text within NCI products is free of copyright and may be reused without our permission. Credit the National Cancer Institute as the source. | |
Record name | Tranexamic acid | |
Source | EPA DSSTox | |
URL | https://comptox.epa.gov/dashboard/DTXSID3045350 | |
Description | DSSTox provides a high quality public chemistry resource for supporting improved predictive toxicology. | |
Record name | 4-(Aminomethyl)-cyclohexanecarboxylic acid | |
Source | EPA DSSTox | |
URL | https://comptox.epa.gov/dashboard/DTXSID50904827 | |
Description | DSSTox provides a high quality public chemistry resource for supporting improved predictive toxicology. | |
Record name | Tranexamic acid | |
Source | European Chemicals Agency (ECHA) | |
URL | https://echa.europa.eu/substance-information/-/substanceinfo/100.013.471 | |
Description | The European Chemicals Agency (ECHA) is an agency of the European Union which is the driving force among regulatory authorities in implementing the EU's groundbreaking chemicals legislation for the benefit of human health and the environment as well as for innovation and competitiveness. | |
Explanation | Use of the information, documents and data from the ECHA website is subject to the terms and conditions of this Legal Notice, and subject to other binding limitations provided for under applicable law, the information, documents and data made available on the ECHA website may be reproduced, distributed and/or used, totally or in part, for non-commercial purposes provided that ECHA is acknowledged as the source: "Source: European Chemicals Agency, http://echa.europa.eu/". Such acknowledgement must be included in each copy of the material. ECHA permits and encourages organisations and individuals to create links to the ECHA website under the following cumulative conditions: Links can only be made to webpages that provide a link to the Legal Notice page. | |
Record name | 4-(Aminomethyl)-cyclohexanecarboxylic acid | |
Source | European Chemicals Agency (ECHA) | |
URL | https://echa.europa.eu/information-on-chemicals | |
Description | The European Chemicals Agency (ECHA) is an agency of the European Union which is the driving force among regulatory authorities in implementing the EU's groundbreaking chemicals legislation for the benefit of human health and the environment as well as for innovation and competitiveness. | |
Explanation | Use of the information, documents and data from the ECHA website is subject to the terms and conditions of this Legal Notice, and subject to other binding limitations provided for under applicable law, the information, documents and data made available on the ECHA website may be reproduced, distributed and/or used, totally or in part, for non-commercial purposes provided that ECHA is acknowledged as the source: "Source: European Chemicals Agency, http://echa.europa.eu/". Such acknowledgement must be included in each copy of the material. ECHA permits and encourages organisations and individuals to create links to the ECHA website under the following cumulative conditions: Links can only be made to webpages that provide a link to the Legal Notice page. | |
Record name | TRANEXAMIC ACID | |
Source | FDA Global Substance Registration System (GSRS) | |
URL | https://gsrs.ncats.nih.gov/ginas/app/beta/substances/6T84R30KC1 | |
Description | The FDA Global Substance Registration System (GSRS) enables the efficient and accurate exchange of information on what substances are in regulated products. Instead of relying on names, which vary across regulatory domains, countries, and regions, the GSRS knowledge base makes it possible for substances to be defined by standardized, scientific descriptions. | |
Explanation | Unless otherwise noted, the contents of the FDA website (www.fda.gov), both text and graphics, are not copyrighted. They are in the public domain and may be republished, reprinted and otherwise used freely by anyone without the need to obtain permission from FDA. Credit to the U.S. Food and Drug Administration as the source is appreciated but not required. | |
Record name | TRANEXAMIC ACID, CIS- | |
Source | FDA Global Substance Registration System (GSRS) | |
URL | https://gsrs.ncats.nih.gov/ginas/app/beta/substances/37YD696II6 | |
Description | The FDA Global Substance Registration System (GSRS) enables the efficient and accurate exchange of information on what substances are in regulated products. Instead of relying on names, which vary across regulatory domains, countries, and regions, the GSRS knowledge base makes it possible for substances to be defined by standardized, scientific descriptions. | |
Explanation | Unless otherwise noted, the contents of the FDA website (www.fda.gov), both text and graphics, are not copyrighted. They are in the public domain and may be republished, reprinted and otherwise used freely by anyone without the need to obtain permission from FDA. Credit to the U.S. Food and Drug Administration as the source is appreciated but not required. | |
Melting Point |
>300 °C | |
Record name | Tranexamic acid | |
Source | DrugBank | |
URL | https://www.drugbank.ca/drugs/DB00302 | |
Description | The DrugBank database is a unique bioinformatics and cheminformatics resource that combines detailed drug (i.e. chemical, pharmacological and pharmaceutical) data with comprehensive drug target (i.e. sequence, structure, and pathway) information. | |
Explanation | Creative Common's Attribution-NonCommercial 4.0 International License (http://creativecommons.org/licenses/by-nc/4.0/legalcode) | |
Synthesis routes and methods
Procedure details
Retrosynthesis Analysis
AI-Powered Synthesis Planning: Our tool employs the Template_relevance Pistachio, Template_relevance Bkms_metabolic, Template_relevance Pistachio_ringbreaker, Template_relevance Reaxys, Template_relevance Reaxys_biocatalysis model, leveraging a vast database of chemical reactions to predict feasible synthetic routes.
One-Step Synthesis Focus: Specifically designed for one-step synthesis, it provides concise and direct routes for your target compounds, streamlining the synthesis process.
Accurate Predictions: Utilizing the extensive PISTACHIO, BKMS_METABOLIC, PISTACHIO_RINGBREAKER, REAXYS, REAXYS_BIOCATALYSIS database, our tool offers high-accuracy predictions, reflecting the latest in chemical research and data.
Strategy Settings
Precursor scoring | Relevance Heuristic |
---|---|
Min. plausibility | 0.01 |
Model | Template_relevance |
Template Set | Pistachio/Bkms_metabolic/Pistachio_ringbreaker/Reaxys/Reaxys_biocatalysis |
Top-N result to add to graph | 6 |
Feasible Synthetic Routes
Q & A
Q1: How does Tranexamic acid exert its hemostatic effect?
A1: Tranexamic acid (trans-4-(aminomethyl)cyclohexanecarboxylic acid) is a synthetic lysine analog that competitively inhibits the activation of plasminogen to plasmin. [] Plasmin is a key enzyme responsible for the breakdown of fibrin clots, which are essential for hemostasis. By blocking plasmin formation, Tranexamic acid prevents clot degradation and promotes blood clotting, thereby reducing blood loss. [, ]
Q2: What is the molecular formula, weight, and any relevant spectroscopic data for Tranexamic acid?
A2: The molecular formula for Tranexamic acid is C8H15NO2. It has a molecular weight of 157.21 g/mol. While the provided research papers do not delve into detailed spectroscopic data, this information can be found in chemical databases and publications focused on the structural characterization of Tranexamic acid.
Q3: The research mentions Tranexamic acid's use in various surgical procedures. How does its stability and material compatibility contribute to these applications?
A3: Tranexamic acid demonstrates good stability under various conditions, allowing for diverse administration routes. It can be administered intravenously, orally, topically, or as an intra-articular injection. [, , , , , , ] Its compatibility with various bodily fluids and tissues, coupled with its stability profile, makes it suitable for these diverse applications.
Q4: Can Tranexamic acid be considered a catalyst? Does it participate in any specific reaction mechanisms?
A4: Tranexamic acid acts as an inhibitor rather than a catalyst. It does not catalyze reactions but instead, binds to the lysine-binding sites on plasminogen, preventing its conversion to plasmin. [] This competitive inhibition mechanism effectively reduces fibrinolysis without directly participating in the catalytic breakdown of fibrin.
Q5: Has computational chemistry been used to study Tranexamic acid? What insights have these studies provided?
A5: While the provided research articles primarily focus on clinical trials and observational studies, computational chemistry methods like molecular docking and QSAR (Quantitative Structure-Activity Relationship) modeling can be valuable tools to investigate the binding interactions of Tranexamic acid with plasminogen and explore potential structural modifications for enhanced efficacy.
Q6: How do structural modifications of Tranexamic acid impact its activity and potency?
A6: While the provided research does not explore specific structural modifications of Tranexamic acid, research in medicinal chemistry can shed light on how alterations to the molecule's structure might influence its binding affinity to plasminogen, potentially impacting its potency, selectivity, and pharmacokinetic properties.
Q7: What are the common formulations of Tranexamic acid, and how do these formulations influence its stability, solubility, and bioavailability?
A7: Tranexamic acid is available in various formulations, including solutions for injection and oral tablets. [] The specific formulation influences its pharmacokinetic properties. For example, intravenous administration leads to rapid systemic distribution, while oral administration requires absorption and may have lower bioavailability. [, ]
Q8: How does the use of Tranexamic acid comply with Safety, Health, and Environment (SHE) regulations?
A8: As a pharmaceutical agent, Tranexamic acid is subject to rigorous SHE regulations during its development, manufacturing, and distribution. [] These regulations ensure the quality, safety, and efficacy of the drug product, minimizing risks to patients and healthcare providers.
Q9: What is the pharmacokinetic (PK) and pharmacodynamic (PD) profile of Tranexamic acid?
A9: Tranexamic acid exhibits rapid absorption following oral administration, with peak plasma concentrations reached within 3 hours. [] It distributes widely into tissues, including the placenta, and is primarily excreted unchanged in the urine. [] Its pharmacodynamic effect is primarily mediated by the inhibition of fibrinolysis, resulting in reduced blood loss. []
Q10: What preclinical models have been employed to study the efficacy and safety of Tranexamic acid?
A10: While not extensively covered in the provided research, preclinical studies using animal models of hemorrhage are crucial for evaluating the efficacy and safety of Tranexamic acid before proceeding to clinical trials. These studies help determine effective dosages, assess potential toxicity, and elucidate the drug's mechanism of action in vivo.
Q11: Are there any specific drug delivery strategies being explored to enhance the targeted delivery of Tranexamic acid?
A11: While the provided research does not focus on novel drug delivery strategies, researchers are constantly exploring targeted drug delivery systems to improve the efficacy and safety of medications. These systems aim to deliver Tranexamic acid more efficiently to the site of bleeding, potentially reducing systemic side effects.
Q12: Are there any biomarkers being investigated to predict the efficacy of Tranexamic acid or to monitor treatment response?
A12: Research on biomarkers for Tranexamic acid is an active area of investigation. Identifying biomarkers could help clinicians personalize treatment strategies, predict patient response, and potentially monitor the drug's efficacy in real-time.
Q13: What analytical methods are commonly employed for the characterization and quantification of Tranexamic acid in biological samples?
A15: High-performance liquid chromatography (HPLC) coupled with various detection methods, such as ultraviolet (UV) or mass spectrometry (MS), is widely used for the accurate quantification of Tranexamic acid in biological matrices. [] These methods allow researchers to study the drug's pharmacokinetic properties and assess its concentration in different tissues.
Q14: What is the environmental impact of Tranexamic acid, and are there any strategies for its degradation or removal from the environment?
A14: While the research provided does not delve into environmental impacts, understanding the fate and effects of pharmaceuticals in the environment is crucial. Further studies are needed to assess the potential ecotoxicological effects of Tranexamic acid and explore strategies for its mitigation.
Q15: How does the dissolution rate of Tranexamic acid tablets influence its bioavailability and efficacy?
A17: The dissolution rate of oral Tranexamic acid tablets is a critical factor influencing its absorption and bioavailability. [] Faster dissolution generally leads to quicker absorption and onset of action. Pharmaceutical scientists optimize tablet formulations to achieve desired dissolution profiles.
Q16: Does Tranexamic acid interact with drug transporters, and if so, what are the implications for its pharmacokinetics?
A21: Tranexamic acid's interaction with drug transporters can influence its absorption, distribution, and elimination. [] Understanding these interactions is crucial for predicting potential drug-drug interactions and optimizing dosing regimens.
Q17: Are there any alternatives or substitutes for Tranexamic acid in clinical practice, and how do they compare in terms of efficacy, safety, and cost?
A24: Other antifibrinolytic drugs, such as aminocaproic acid, can be considered as alternatives to Tranexamic acid in certain clinical scenarios. [] The choice of agent depends on factors such as the specific clinical indication, patient characteristics, cost considerations, and potential adverse effect profiles.
Q18: What research infrastructure and resources are crucial for advancing our understanding of Tranexamic acid and its applications?
A18: Continued research on Tranexamic acid requires a robust infrastructure encompassing clinical trial networks, biobanks, and access to advanced analytical techniques. Collaboration between clinicians, scientists, and pharmaceutical companies is vital for driving innovation and translating research findings into clinical practice.
Q19: What are some historical milestones in the research and development of Tranexamic acid as a hemostatic agent?
A27: The discovery of Tranexamic acid's antifibrinolytic properties and its subsequent development as a hemostatic agent represent significant milestones in medicine. [] Over the years, large-scale clinical trials, such as the WOMAN trial, have provided robust evidence supporting its efficacy in reducing blood loss during surgery and childbirth. [, ]
Disclaimer and Information on In-Vitro Research Products
Please be aware that all articles and product information presented on BenchChem are intended solely for informational purposes. The products available for purchase on BenchChem are specifically designed for in-vitro studies, which are conducted outside of living organisms. In-vitro studies, derived from the Latin term "in glass," involve experiments performed in controlled laboratory settings using cells or tissues. It is important to note that these products are not categorized as medicines or drugs, and they have not received approval from the FDA for the prevention, treatment, or cure of any medical condition, ailment, or disease. We must emphasize that any form of bodily introduction of these products into humans or animals is strictly prohibited by law. It is essential to adhere to these guidelines to ensure compliance with legal and ethical standards in research and experimentation.