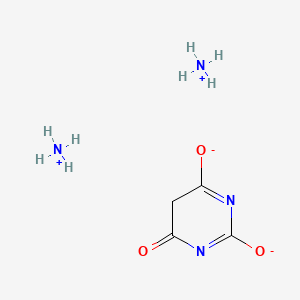
Ammonium barbiturate
- Click on QUICK INQUIRY to receive a quote from our team of experts.
- With the quality product at a COMPETITIVE price, you can focus more on your research.
Overview
Description
Ammonium barbiturate is a chemical compound derived from barbituric acid. Barbiturates are a class of central nervous system depressants that were first synthesized in the early 20th century. They have been used for various medical purposes, including as sedatives, hypnotics, and anticonvulsants . This compound, specifically, is formed by the reaction of barbituric acid with ammonia, resulting in a crystalline salt.
Preparation Methods
Synthetic Routes and Reaction Conditions
Ammonium barbiturate can be synthesized through gas-solid reactions between barbituric acid and ammonia. The reaction involves exposing barbituric acid to ammonia gas, leading to the formation of this compound crystals . The reaction conditions typically include maintaining a controlled temperature and pressure to ensure the complete conversion of barbituric acid to this compound.
Industrial Production Methods
Industrial production of this compound follows similar principles but on a larger scale. The process involves the continuous flow of ammonia gas over barbituric acid in a reactor. The reaction is monitored to maintain optimal conditions, and the resulting this compound is collected and purified for further use.
Chemical Reactions Analysis
Types of Reactions
Ammonium barbiturate undergoes various chemical reactions, including:
Substitution Reactions: It can participate in nucleophilic substitution reactions where the ammonium ion is replaced by other cations.
Dehydration Reactions: Heating this compound can lead to the loss of ammonia, reverting it back to barbituric acid.
Common Reagents and Conditions
Common reagents used in reactions with this compound include acids and bases, which can facilitate the substitution and dehydration processes. The reactions are typically carried out under controlled temperatures to ensure the desired products are formed.
Major Products Formed
The major products formed from the reactions of this compound include substituted barbiturates and barbituric acid, depending on the specific reaction conditions and reagents used.
Scientific Research Applications
Ammonium barbiturate has several scientific research applications:
Biology: Researchers use this compound to study its effects on biological systems, particularly its interactions with enzymes and receptors.
Industry: In industrial settings, this compound is used in the production of other barbiturate compounds and as an intermediate in chemical synthesis.
Mechanism of Action
Ammonium barbiturate exerts its effects by interacting with the gamma-aminobutyric acid (GABA) receptor in the central nervous system. It binds to the GABA_A receptor, enhancing the inhibitory effects of GABA, which leads to a calming effect on the brain . This mechanism is similar to other barbiturates, which also potentiate the effect of GABA at this receptor.
Comparison with Similar Compounds
Similar Compounds
Similar compounds to ammonium barbiturate include:
Phenobarbital: A long-acting barbiturate used as an anticonvulsant.
Pentobarbital: A short-acting barbiturate used for sedation and anesthesia.
Secobarbital: A short-acting barbiturate used as a sedative and hypnotic.
Uniqueness
This compound is unique in its formation and specific applications. Unlike other barbiturates, it is primarily used as an intermediate in chemical synthesis rather than as a direct therapeutic agent. Its ability to form crystalline salts with ammonia also distinguishes it from other barbiturates.
Biological Activity
Ammonium barbiturate, a derivative of barbituric acid, is a compound that has been studied for its various biological activities. This article provides a comprehensive overview of its biological properties, including antibacterial, sedative, and enzyme inhibition effects, supported by relevant case studies and research findings.
Overview of Barbiturates
Barbiturates, including this compound, are a class of drugs that act as central nervous system depressants. They are primarily known for their sedative and hypnotic properties, historically used in the treatment of anxiety, insomnia, and seizures. Despite the rise of benzodiazepines, barbiturates remain significant in medicinal chemistry due to their diverse biological activities.
1. Sedative and Hypnotic Effects
Barbiturates exert their sedative effects primarily through the modulation of gamma-aminobutyric acid (GABA) receptors in the brain. They enhance GABA's inhibitory action, leading to decreased neuronal excitability. This mechanism is crucial for their use in anesthesia and the management of sleep disorders. Research indicates that this compound binds to distinct sites on the GABA_A receptor, which enhances chloride ion influx and results in hyperpolarization of neurons .
2. Antibacterial Activity
Recent studies have highlighted the antibacterial properties of various barbiturate derivatives. For example, arylidene barbiturates have shown significant antibacterial activity influenced by structural modifications such as the presence of hydroxyl groups in specific positions on the aryl chain. These modifications enhance the compounds' efficacy against a range of bacterial strains .
Table 1: Antibacterial Activity of Selected Barbiturate Derivatives
Compound Name | Minimum Inhibitory Concentration (MIC) | Bacterial Strain Tested |
---|---|---|
Arylidene Barbiturate A | 12 µg/mL | Staphylococcus aureus |
Arylidene Barbiturate B | 25 µg/mL | Escherichia coli |
Hydroxy-substituted Barbiturate C | 8 µg/mL | Pseudomonas aeruginosa |
3. Enzyme Inhibition
This compound has been investigated for its potential as an enzyme inhibitor. Studies have shown that certain derivatives can inhibit enzymes such as xanthine oxidase and α-glucosidase, which are relevant in conditions like gout and diabetes . The inhibitory effects are often linked to specific structural features within the barbiturate framework.
Table 2: Enzyme Inhibition Potency of Barbiturate Derivatives
Compound Name | IC50 (µM) | Enzyme Target |
---|---|---|
Compound D | 24.3 | Xanthine Oxidase |
Compound E | 27.9 | Xanthine Oxidase |
Compound F | 101.8 | α-Glucosidase |
Case Study 1: Antimicrobial Efficacy
A study focused on synthesizing various arylidene barbiturates demonstrated that compounds with hydroxyl groups at the ortho position exhibited enhanced antibacterial activity against Gram-positive bacteria. The research concluded that structural modifications could significantly influence antimicrobial efficacy .
Case Study 2: Sedative Properties
In clinical settings, this compound has been utilized for its sedative effects during surgical procedures. Its rapid onset and short duration make it suitable for induction anesthesia. However, careful monitoring is required due to its narrow therapeutic index and potential for respiratory depression .
Properties
CAS No. |
67008-01-9 |
---|---|
Molecular Formula |
C4H10N4O3 |
Molecular Weight |
162.15 g/mol |
IUPAC Name |
diazanium;6-oxo-5H-pyrimidine-2,4-diolate |
InChI |
InChI=1S/C4H4N2O3.2H3N/c7-2-1-3(8)6-4(9)5-2;;/h1H2,(H2,5,6,7,8,9);2*1H3 |
InChI Key |
XNDLCVVPLCWBGH-UHFFFAOYSA-N |
Canonical SMILES |
C1C(=NC(=NC1=O)[O-])[O-].[NH4+].[NH4+] |
Origin of Product |
United States |
Disclaimer and Information on In-Vitro Research Products
Please be aware that all articles and product information presented on BenchChem are intended solely for informational purposes. The products available for purchase on BenchChem are specifically designed for in-vitro studies, which are conducted outside of living organisms. In-vitro studies, derived from the Latin term "in glass," involve experiments performed in controlled laboratory settings using cells or tissues. It is important to note that these products are not categorized as medicines or drugs, and they have not received approval from the FDA for the prevention, treatment, or cure of any medical condition, ailment, or disease. We must emphasize that any form of bodily introduction of these products into humans or animals is strictly prohibited by law. It is essential to adhere to these guidelines to ensure compliance with legal and ethical standards in research and experimentation.