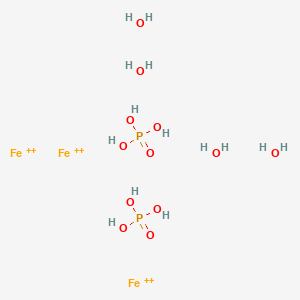
Vivianite
- Click on QUICK INQUIRY to receive a quote from our team of experts.
- With the quality product at a COMPETITIVE price, you can focus more on your research.
Overview
Description
Vivianite is a hydrated iron phosphate mineral with the chemical formula Fe₃(PO₄)₂·8H₂O. It is known for its striking blue to blue-green color, which can change to dark blue or black upon oxidation. This compound is often found in association with iron ore deposits, swamps, bogs, and other water-rich environments. It was named after John Henry Vivian, a British industrialist and mine owner .
Preparation Methods
Synthetic Routes and Reaction Conditions: Vivianite can be synthesized through various methods, including the microbial reduction of ferrihydrite in the presence of phosphate ions. This process involves the use of dissimilatory iron-reducing bacteria, which facilitate the reduction of ferric iron to ferrous iron, leading to the formation of this compound .
Industrial Production Methods: In industrial settings, this compound can be produced from anaerobically digested sewage sludge. The addition of magnetic biochar to the sludge enhances the recovery of phosphorus as this compound. The process involves hydrothermal pretreatment and anaerobic digestion, optimizing conditions such as digestion time and temperature to maximize this compound formation .
Chemical Reactions Analysis
Types of Reactions: Vivianite undergoes several types of chemical reactions, including oxidation, reduction, and precipitation. The oxidation of this compound involves the conversion of ferrous iron (Fe²⁺) to ferric iron (Fe³⁺), leading to a change in color from blue to dark blue or black .
Common Reagents and Conditions:
Oxidation: Exposure to oxygen or other oxidizing agents.
Reduction: Microbial reduction using dissimilatory iron-reducing bacteria.
Precipitation: Formation in the presence of phosphate ions under anaerobic conditions.
Major Products Formed:
Oxidation: Metathis compound (oxidized form of this compound).
Reduction: Formation of this compound from ferrihydrite and phosphate ions
Scientific Research Applications
Vivianite has a wide range of scientific research applications across various fields:
Chemistry:
Phosphorus Recovery: this compound is used to recover phosphorus from wastewater, addressing both phosphorus shortage and pollution.
Biology:
Microbial Studies: The formation of this compound through microbial reduction provides insights into microbial iron reduction processes.
Medicine:
Forensic Science: this compound encrustation on skeletal remains can provide information about burial environments and post-mortem changes.
Industry:
Mechanism of Action
The mechanism of action of vivianite involves its dissolution and oxidation processes. Under anoxic conditions, this compound dissolves through a defect-driven mechanism, with dissolution rates influenced by pH and temperature. The oxidation of ferrous iron (Fe²⁺) in this compound involves the conversion of water ligands to hydroxide ions, leading to the collapse of the this compound structure and further oxidation .
Comparison with Similar Compounds
- Pakhomovskyite (Co₃(PO₄)₂·8H₂O)
- Erythrite (Co₃(AsO₄)₂·8H₂O)
Vivianite’s unique properties and diverse applications make it a valuable compound in various scientific and industrial fields.
Biological Activity
Vivianite, a hydrated iron phosphate mineral with the formula Fe32+(PO4)2·8H2O, has garnered attention for its biological significance, particularly in biogeochemical cycles and its potential applications in environmental and agricultural contexts. This article discusses the biological activity of this compound, focusing on its formation, dissolution mechanisms, and interactions with microorganisms.
1. Formation and Biosynthesis of this compound
This compound formation is primarily influenced by microbial activity in anoxic environments. The process involves dissimilatory iron reduction (DIR), where microorganisms reduce Fe(III) to Fe(II), facilitating the precipitation of this compound from iron oxides.
Key Factors Influencing this compound Formation:
- Microorganisms : Specific bacteria, such as Geobacter sulfurreducens, play a crucial role in the reduction of iron oxides, leading to this compound biosynthesis .
- Environmental Conditions : Factors such as pH, temperature, and the Fe/P molar ratio significantly affect the biosynthesis process .
- Crystal Surface Structure : The morphology of iron oxides influences microbial attachment and reduction rates, impacting this compound formation .
2. Dissolution Mechanisms
Understanding the dissolution kinetics of this compound is essential for assessing its role as a phosphorus source in various environments. Research indicates that the dissolution rates of this compound are highly dependent on pH levels.
Dissolution Characteristics:
- pH Influence : this compound solubility increases significantly at lower pH levels. For instance, at pH 5, complete dissolution occurs rapidly, while at pH 9, solubility decreases markedly .
- Non-Stoichiometric Dissolution : The dissolution process is non-stoichiometric under alkaline conditions, leading to varying concentrations of dissolved iron and phosphate ions .
3. Biological Interactions
This compound's interaction with microorganisms highlights its potential as an energy source in biogeochemical processes. Studies have demonstrated that certain acidophilic bacteria can utilize this compound as an energy source.
Microbial Utilization:
- Acidithiobacillus ferrooxidans : This bacterium can oxidize Fe2+ derived from this compound under low pH conditions, showcasing its ability to thrive on minerals like this compound and siderite .
4. Case Studies
Several studies illustrate the biological activity of this compound in different contexts:
Case Study 1: Microbial Reduction and this compound Formation
A study conducted using G. sulfurreducens demonstrated that under controlled laboratory conditions, the reduction of iron oxides led to significant this compound formation over a 30-day period. The study highlighted how varying electron donors affected microbial activity and subsequent mineral precipitation .
Case Study 2: Environmental Impact on Phosphorus Cycling
Research focusing on anaerobic digesters revealed that high concentrations of this compound enhance iron mobility in phosphorus-rich environments. This mobility facilitates better nutrient cycling and availability for microbial communities .
5. Data Summary
The following table summarizes key findings related to the biological activity of this compound:
Properties
CAS No. |
14567-67-0 |
---|---|
Molecular Formula |
Fe3H14O12P2+6 |
Molecular Weight |
435.59 g/mol |
IUPAC Name |
iron(2+);phosphoric acid;tetrahydrate |
InChI |
InChI=1S/3Fe.2H3O4P.4H2O/c;;;2*1-5(2,3)4;;;;/h;;;2*(H3,1,2,3,4);4*1H2/q3*+2;;;;;; |
InChI Key |
XUGKISOGTYVYEG-UHFFFAOYSA-N |
Canonical SMILES |
O.O.O.O.OP(=O)(O)O.OP(=O)(O)O.[Fe+2].[Fe+2].[Fe+2] |
Origin of Product |
United States |
Disclaimer and Information on In-Vitro Research Products
Please be aware that all articles and product information presented on BenchChem are intended solely for informational purposes. The products available for purchase on BenchChem are specifically designed for in-vitro studies, which are conducted outside of living organisms. In-vitro studies, derived from the Latin term "in glass," involve experiments performed in controlled laboratory settings using cells or tissues. It is important to note that these products are not categorized as medicines or drugs, and they have not received approval from the FDA for the prevention, treatment, or cure of any medical condition, ailment, or disease. We must emphasize that any form of bodily introduction of these products into humans or animals is strictly prohibited by law. It is essential to adhere to these guidelines to ensure compliance with legal and ethical standards in research and experimentation.