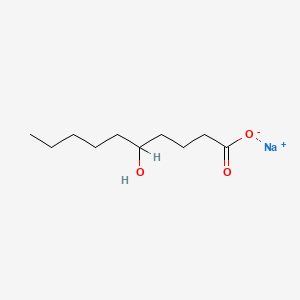
Sodium 5-hydroxydecanoate
Overview
Description
5-Hydroxydecanoic acid sodium is a chemical compound with the molecular formula C10H19NaO3 and a molecular weight of 210.25 g/mol . It is a sodium salt derivative of 5-hydroxydecanoic acid, characterized by its white to beige powder form and high solubility in water . This compound is known for its role as a potassium channel antagonist, particularly in blocking the actions of potassium channel activators during ischemic conditions .
Mechanism of Action
Target of Action
Sodium 5-Hydroxydecanoate, also known as 5-Hydroxydecanoic acid sodium salt, primarily targets the mitochondrial ATP-sensitive potassium (KATP) channels . These channels play a crucial role in cellular energy metabolism and are involved in various physiological processes, including insulin secretion, vascular tone regulation, and protection against ischemic injury .
Mode of Action
This compound acts as a selective blocker of the KATP channels . By inhibiting these channels, it prevents the efflux of potassium ions, which can affect the membrane potential and cellular excitability . This compound is also a substrate for mitochondrial outer membrane acyl-CoA synthetase .
Biochemical Pathways
The inhibition of KATP channels by this compound can impact several biochemical pathways. For instance, it can inhibit the proliferation of hypoxic human pulmonary artery smooth muscle cells (HPASMC) by blocking mitochondrial KATP channels . This suggests a role in the regulation of cell proliferation under hypoxic conditions.
Result of Action
The blockade of KATP channels by this compound can lead to various cellular effects. For example, it has been shown to inhibit the post-ischemic actions of the KATP channel activator cromakalim . Moreover, it can inhibit the proliferation of HPASMC, suggesting potential implications in conditions like pulmonary hypertension .
Biochemical Analysis
Biochemical Properties
Sodium 5-hydroxydecanoate plays a significant role in biochemical reactions by inhibiting mitochondrial KATP channels. This inhibition affects the mitochondrial membrane potential and ATP production. This compound interacts with various enzymes and proteins, including acyl-CoA synthetase and potassium channels. The nature of these interactions involves the binding of this compound to the active sites of these enzymes, leading to inhibition or modulation of their activity .
Cellular Effects
This compound has profound effects on various types of cells and cellular processes. It influences cell function by modulating cell signaling pathways, gene expression, and cellular metabolism. For instance, in hypoxic human pulmonary artery smooth muscle cells, this compound inhibits proliferation by blocking mitochondrial KATP channels . Additionally, it affects ATP-depleted renal epithelial cells by increasing cytotoxicity and apoptosis through superoxide-dependent mechanisms .
Molecular Mechanism
The molecular mechanism of this compound involves its action as a mitochondrial KATP channel inhibitor. By blocking these channels, this compound prevents the efflux of potassium ions, thereby maintaining the mitochondrial membrane potential. This action leads to the inhibition of ATP production and affects various cellular processes. This compound also interacts with other biomolecules, such as Na+/K±ATPase, further influencing cellular functions .
Temporal Effects in Laboratory Settings
In laboratory settings, the effects of this compound change over time. The compound is stable under desiccated conditions and can be stored for up to 12 months at -20°C . Long-term exposure to this compound in in vitro studies has shown increased cytotoxicity and apoptosis in ATP-depleted cells . These temporal effects highlight the importance of considering the duration of exposure when studying the compound’s effects.
Dosage Effects in Animal Models
The effects of this compound vary with different dosages in animal models. In studies involving paclitaxel-induced neurotoxicity, this compound exhibited neuroprotective effects at specific dosages . At higher doses, the compound may exhibit toxic or adverse effects, emphasizing the need for careful dosage optimization in experimental settings.
Metabolic Pathways
This compound is involved in various metabolic pathways, particularly those related to fatty acid metabolism. It interacts with enzymes such as acyl-CoA synthetase, which plays a role in the activation of fatty acids for subsequent metabolic processes . The compound’s involvement in these pathways can influence metabolic flux and metabolite levels, impacting overall cellular metabolism.
Transport and Distribution
Within cells and tissues, this compound is transported and distributed through interactions with specific transporters and binding proteins. It is a substrate for mitochondrial outer membrane acyl-CoA synthetase, which facilitates its transport into mitochondria . This localization is crucial for its function as a mitochondrial KATP channel inhibitor.
Subcellular Localization
This compound is primarily localized in the mitochondria, where it exerts its inhibitory effects on KATP channels. This subcellular localization is directed by targeting signals and post-translational modifications that ensure its proper distribution within the cell . The mitochondrial localization is essential for its role in modulating mitochondrial function and cellular metabolism.
Preparation Methods
Synthetic Routes and Reaction Conditions: The synthesis of 5-hydroxydecanoic acid sodium typically involves the hydroxylation of decanoic acid followed by neutralization with sodium hydroxide. The reaction conditions often include:
Hydroxylation: Decanoic acid is subjected to hydroxylation using a suitable hydroxylating agent under controlled temperature and pressure conditions.
Neutralization: The resulting 5-hydroxydecanoic acid is then neutralized with sodium hydroxide to form the sodium salt.
Industrial Production Methods: In industrial settings, the production of 5-hydroxydecanoic acid sodium may involve large-scale hydroxylation reactors and continuous neutralization processes to ensure consistent quality and yield. The compound is then purified through crystallization and drying processes to obtain the final product .
Chemical Reactions Analysis
Types of Reactions: 5-Hydroxydecanoic acid sodium undergoes various chemical reactions, including:
Oxidation: The hydroxyl group can be oxidized to form a ketone or carboxylic acid.
Reduction: The carboxylate group can be reduced to form an alcohol.
Substitution: The hydroxyl group can participate in nucleophilic substitution reactions.
Common Reagents and Conditions:
Oxidation: Common oxidizing agents include potassium permanganate and chromium trioxide.
Reduction: Reducing agents such as lithium aluminum hydride or sodium borohydride are used.
Substitution: Nucleophiles like halides or amines can be used under basic or acidic conditions.
Major Products:
Oxidation: Formation of 5-ketodecanoic acid or 5-carboxydecanoic acid.
Reduction: Formation of 5-hydroxydecanoic alcohol.
Substitution: Formation of various substituted decanoic acid derivatives.
Scientific Research Applications
5-Hydroxydecanoic acid sodium has a wide range of applications in scientific research:
Chemistry: Used as a reagent in organic synthesis and as a standard in analytical chemistry.
Biology: Studied for its role in cellular metabolism and signaling pathways.
Medicine: Investigated for its potential therapeutic effects in cardiovascular diseases due to its potassium channel blocking properties.
Comparison with Similar Compounds
5-Hydroxydecanoic acid: The parent compound without the sodium salt.
10-Hydroxydecanoic acid: A similar compound with the hydroxyl group at a different position.
5-Ketodecanoic acid: An oxidized derivative of 5-hydroxydecanoic acid.
Uniqueness: 5-Hydroxydecanoic acid sodium is unique due to its specific action on potassium channels and its enhanced solubility in water compared to its parent compound. This makes it particularly useful in biological and medical research where aqueous solubility is essential .
Properties
IUPAC Name |
sodium;5-hydroxydecanoate | |
---|---|---|
Source | PubChem | |
URL | https://pubchem.ncbi.nlm.nih.gov | |
Description | Data deposited in or computed by PubChem | |
InChI |
InChI=1S/C10H20O3.Na/c1-2-3-4-6-9(11)7-5-8-10(12)13;/h9,11H,2-8H2,1H3,(H,12,13);/q;+1/p-1 | |
Source | PubChem | |
URL | https://pubchem.ncbi.nlm.nih.gov | |
Description | Data deposited in or computed by PubChem | |
InChI Key |
YNAGNECWEKMWRM-UHFFFAOYSA-M | |
Source | PubChem | |
URL | https://pubchem.ncbi.nlm.nih.gov | |
Description | Data deposited in or computed by PubChem | |
Canonical SMILES |
CCCCCC(CCCC(=O)[O-])O.[Na+] | |
Source | PubChem | |
URL | https://pubchem.ncbi.nlm.nih.gov | |
Description | Data deposited in or computed by PubChem | |
Molecular Formula |
C10H19NaO3 | |
Source | PubChem | |
URL | https://pubchem.ncbi.nlm.nih.gov | |
Description | Data deposited in or computed by PubChem | |
DSSTOX Substance ID |
DTXSID101017457 | |
Record name | 5-Hydroxydecanoic acid sodium salt | |
Source | EPA DSSTox | |
URL | https://comptox.epa.gov/dashboard/DTXSID101017457 | |
Description | DSSTox provides a high quality public chemistry resource for supporting improved predictive toxicology. | |
Molecular Weight |
210.25 g/mol | |
Source | PubChem | |
URL | https://pubchem.ncbi.nlm.nih.gov | |
Description | Data deposited in or computed by PubChem | |
CAS No. |
71186-53-3 | |
Record name | 5-Hydroxydecanoic acid sodium salt | |
Source | EPA DSSTox | |
URL | https://comptox.epa.gov/dashboard/DTXSID101017457 | |
Description | DSSTox provides a high quality public chemistry resource for supporting improved predictive toxicology. | |
Retrosynthesis Analysis
AI-Powered Synthesis Planning: Our tool employs the Template_relevance Pistachio, Template_relevance Bkms_metabolic, Template_relevance Pistachio_ringbreaker, Template_relevance Reaxys, Template_relevance Reaxys_biocatalysis model, leveraging a vast database of chemical reactions to predict feasible synthetic routes.
One-Step Synthesis Focus: Specifically designed for one-step synthesis, it provides concise and direct routes for your target compounds, streamlining the synthesis process.
Accurate Predictions: Utilizing the extensive PISTACHIO, BKMS_METABOLIC, PISTACHIO_RINGBREAKER, REAXYS, REAXYS_BIOCATALYSIS database, our tool offers high-accuracy predictions, reflecting the latest in chemical research and data.
Strategy Settings
Precursor scoring | Relevance Heuristic |
---|---|
Min. plausibility | 0.01 |
Model | Template_relevance |
Template Set | Pistachio/Bkms_metabolic/Pistachio_ringbreaker/Reaxys/Reaxys_biocatalysis |
Top-N result to add to graph | 6 |
Feasible Synthetic Routes
Q1: What is the primary target of Sodium 5-hydroxydecanoate?
A1: this compound acts as an antagonist of ATP-sensitive potassium (KATP) channels. [, , , , , , , , , , , , , , , , , , , , , , , , , , , ]
Q2: How does blocking KATP channels with 5-HD impact the heart during ischemia?
A2: KATP channels open during ischemia when ATP levels fall. By blocking these channels, 5-HD prevents potassium efflux, potentially influencing action potential duration, cellular excitability, and ultimately impacting the extent of ischemic damage. [, , , ]
Q3: Is this compound considered a selective KATP channel blocker?
A3: While 5-HD effectively blocks KATP channels, research suggests it might have some selectivity towards mitochondrial KATP channels over those located on the cell surface (sarcolemmal). [, , , , , , , , , , ]
Q4: Does this compound directly affect cardiac contractility?
A4: Studies indicate that 5-HD, at concentrations typically used to block KATP channels, does not directly impact cardiac contractile force. [, ]
Q5: What is the molecular formula and weight of this compound?
A5: The molecular formula of this compound is C10H19NaO3, and its molecular weight is 214.26 g/mol.
Q6: Is there information available on the spectroscopic characteristics of 5-HD?
A6: The provided research papers do not delve into detailed spectroscopic analyses of 5-HD.
Q7: How is this compound used to study ischemic preconditioning?
A7: Ischemic preconditioning is a phenomenon where brief episodes of ischemia protect the heart from subsequent, more prolonged ischemic insults. Researchers use 5-HD to investigate whether the opening of KATP channels is essential for this protective effect. If 5-HD abolishes the benefits of preconditioning, it suggests a critical role for KATP channels. [, , , , , , , , ]
Q8: Has this compound been used to explore the role of KATP channels in the cardioprotective effects of other agents?
A8: Yes, 5-HD has been used to investigate whether the cardioprotective effects of various drugs, including volatile anesthetics like isoflurane and pharmacological agents like geranylgeranylacetone, are mediated by KATP channel opening. [, ]
Q9: Is this compound being developed as a therapeutic drug?
A10: this compound is primarily a research tool. Its use in clinical settings is limited due to its potential for off-target effects and the availability of more selective KATP channel modulators. []
Disclaimer and Information on In-Vitro Research Products
Please be aware that all articles and product information presented on BenchChem are intended solely for informational purposes. The products available for purchase on BenchChem are specifically designed for in-vitro studies, which are conducted outside of living organisms. In-vitro studies, derived from the Latin term "in glass," involve experiments performed in controlled laboratory settings using cells or tissues. It is important to note that these products are not categorized as medicines or drugs, and they have not received approval from the FDA for the prevention, treatment, or cure of any medical condition, ailment, or disease. We must emphasize that any form of bodily introduction of these products into humans or animals is strictly prohibited by law. It is essential to adhere to these guidelines to ensure compliance with legal and ethical standards in research and experimentation.