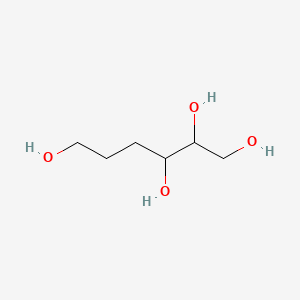
Hexane-1,2,3,6-tetrol
- Click on QUICK INQUIRY to receive a quote from our team of experts.
- With the quality product at a COMPETITIVE price, you can focus more on your research.
Overview
Description
Hexane-1,2,3,6-tetrol is an organic compound with the molecular formula C6H14O4 It is a tetrol, meaning it contains four hydroxyl (OH) groups attached to a hexane backbone
Preparation Methods
Synthetic Routes and Reaction Conditions
Hexane-1,2,3,6-tetrol can be synthesized through several methods. One common approach involves the catalytic hydrogenation of hexane derivatives. For instance, the hydrogenation of hexane-1,2,3,6-tetraone in the presence of a platinum catalyst can yield this compound. The reaction typically occurs under mild conditions, such as room temperature and atmospheric pressure.
Industrial Production Methods
In an industrial setting, this compound can be produced from bio-renewable sources. For example, the catalytic conversion of cellulose-derived levoglucosanol in water using a bifunctional Pt/SiO2-Al2O3 catalyst at 150°C can yield this compound with high efficiency .
Chemical Reactions Analysis
Types of Reactions
Hexane-1,2,3,6-tetrol undergoes various chemical reactions, including:
Oxidation: The hydroxyl groups can be oxidized to form corresponding ketones or aldehydes.
Reduction: The compound can be reduced to form hexane derivatives with fewer hydroxyl groups.
Substitution: The hydroxyl groups can be substituted with other functional groups, such as halogens or alkyl groups.
Common Reagents and Conditions
Oxidation: Common oxidizing agents include potassium permanganate (KMnO4) and chromium trioxide (CrO3).
Reduction: Hydrogen gas (H2) in the presence of a metal catalyst, such as palladium (Pd) or platinum (Pt), is commonly used.
Substitution: Reagents like thionyl chloride (SOCl2) or phosphorus tribromide (PBr3) can be used for halogenation reactions.
Major Products
Oxidation: Hexane-1,2,3,6-tetraone
Reduction: Hexane-1,2,3-triol
Substitution: Hexane-1,2,3,6-tetrahalide
Scientific Research Applications
Hexane-1,2,3,6-tetrol has several applications in scientific research:
Chemistry: It is used as a building block for the synthesis of complex organic molecules and polymers.
Biology: The compound can be used in the study of metabolic pathways involving polyols.
Medicine: this compound derivatives are investigated for their potential therapeutic properties.
Industry: It is used in the production of bio-based chemicals, solvents, and surfactants.
Mechanism of Action
The mechanism by which hexane-1,2,3,6-tetrol exerts its effects depends on its interaction with specific molecular targets. For example, in biological systems, the hydroxyl groups can form hydrogen bonds with enzymes or receptors, influencing their activity. The compound can also participate in redox reactions, altering the oxidative state of cellular components .
Comparison with Similar Compounds
Similar Compounds
- Hexane-1,2,5,6-tetrol
- Hexane-1,2,3,4-tetrol
- Hexane-1,2,3-triol
Uniqueness
Hexane-1,2,3,6-tetrol is unique due to the specific positioning of its hydroxyl groups, which imparts distinct chemical and physical properties. This unique structure allows for specific interactions in chemical reactions and biological systems, making it valuable for targeted applications .
Biological Activity
Hexane-1,2,3,6-tetrol (often referred to as tetrol) is a polyol derived from cellulose and has garnered attention for its potential applications in various fields, including biochemistry and materials science. This article explores the biological activity of this compound, focusing on its synthesis, properties, and implications for health and industry.
This compound can be synthesized through catalytic processes involving levoglucosanol (lgol), a cellulose-derived compound. The synthesis typically involves the following steps:
- Hydrolysis : Lgol undergoes hydrolysis to form intermediates such as 3,4-dideoxymannose (DDM) and 3,4-dideoxyglucose (DDG).
- Isomerization : At elevated temperatures, DDM and DDG can isomerize to 3,4-dideoxyfructose (DDF).
- Hydrogenation : DDM is converted to cis-tetrol while DDG is converted to trans-tetrol through hydrogenation over bifunctional metal catalysts like Pt/SiO₂-Al₂O₃.
The overall yield of tetrol can reach up to 90% under optimal conditions .
Antimicrobial Properties
Recent studies have indicated that this compound exhibits antimicrobial properties. A study assessing its antibacterial activity showed that it could inhibit the growth of various bacterial strains when applied in specific concentrations. The results demonstrated significant zones of inhibition against pathogens such as Escherichia coli and Staphylococcus aureus, suggesting potential applications in pharmaceuticals as a natural preservative or antimicrobial agent .
Cytotoxicity and Safety
The cytotoxicity of this compound has been evaluated using various cell lines. Research indicates that at low concentrations, tetrol does not exhibit significant cytotoxic effects. However, at higher concentrations, it may induce apoptosis in certain cancer cell lines. This dual nature suggests that while it may serve as a therapeutic agent in controlled doses, caution is warranted regarding its concentration in formulations .
Applications in Material Science
This compound is not only relevant in biological contexts but also serves as a versatile building block in material science:
- Polymer Production : Tetrol can be dehydrated to produce polymer precursors such as tetrahydrofuran dimethyl ether (THFDM), which can further be transformed into valuable polymers like polyesters and polyurethanes .
- Chiral Materials : The condensation of this compound with boronic acids leads to the formation of mesoporous chiral polyboronates. These materials have potential applications in catalysis and drug delivery due to their unique structural properties .
Research Findings Summary
The following table summarizes key findings regarding the biological activity and applications of this compound:
Case Study 1: Antimicrobial Efficacy
A controlled study evaluated the antimicrobial efficacy of this compound against common bacterial strains. The results indicated that a concentration of 8 mg/mL resulted in a zone of inhibition averaging 15 mm against E. coli, demonstrating its potential as an antimicrobial agent .
Case Study 2: Application in Drug Delivery Systems
Research exploring the use of this compound-based chiral polyboronates showed promising results in drug delivery systems due to their high surface area and porosity. These materials facilitated enhanced drug loading capacities compared to traditional carriers .
Properties
CAS No. |
84709-11-5 |
---|---|
Molecular Formula |
C6H14O4 |
Molecular Weight |
150.17 g/mol |
IUPAC Name |
hexane-1,2,3,6-tetrol |
InChI |
InChI=1S/C6H14O4/c7-3-1-2-5(9)6(10)4-8/h5-10H,1-4H2 |
InChI Key |
RLMXGBGAZRVYIX-UHFFFAOYSA-N |
Canonical SMILES |
C(CC(C(CO)O)O)CO |
Origin of Product |
United States |
Disclaimer and Information on In-Vitro Research Products
Please be aware that all articles and product information presented on BenchChem are intended solely for informational purposes. The products available for purchase on BenchChem are specifically designed for in-vitro studies, which are conducted outside of living organisms. In-vitro studies, derived from the Latin term "in glass," involve experiments performed in controlled laboratory settings using cells or tissues. It is important to note that these products are not categorized as medicines or drugs, and they have not received approval from the FDA for the prevention, treatment, or cure of any medical condition, ailment, or disease. We must emphasize that any form of bodily introduction of these products into humans or animals is strictly prohibited by law. It is essential to adhere to these guidelines to ensure compliance with legal and ethical standards in research and experimentation.