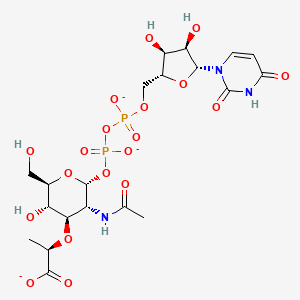
UDP-N-acetylmuramic acid
- Click on QUICK INQUIRY to receive a quote from our team of experts.
- With the quality product at a COMPETITIVE price, you can focus more on your research.
Overview
Description
UDP-N-acetyl-alpha-D-muramate(3-) is a UDP-N-acetyl-D-muramate(3-) in which the anomeric centre of the pyranose fragment has alpha-configuration. It is a conjugate base of an UDP-N-acetyl-alpha-D-muramic acid.
A nucleoside diphosphate sugar which is formed from UDP-N-acetylglucosamine and phosphoenolpyruvate. It serves as the building block upon which peptidoglycan is formed.
Scientific Research Applications
Synthesis Optimization
UDP-N-acetylmuramic acid (UDP-MurNAc) is vital in bacterial peptidoglycan biosynthesis. Humljan et al. (2008) optimized its synthesis, focusing on muramyl phosphate and UMP-morpholidate coupling, enabling reproducible multi-gram scale production (Humljan et al., 2008).
Enzymatic Synthesis
Raymond et al. (2003) developed an enzymatic scheme for UDP-MurNAc synthesis using Escherichia coli enzymes, facilitating its availability for peptidoglycan pathway studies (Raymond, Price, & Pavelka, 2003).
Structural Analysis
Mol et al. (2003) examined Haemophilus influenzae MurC, which catalyzes the addition of the first amino acid to the cytoplasmic precursor of bacterial cell wall peptidoglycan. They determined crystal structures of MurC in complex with UDP-MurNAc, providing insights into its functioning (Mol et al., 2003).
Recycling Pathway in Gram-negative Bacteria
Gisin et al. (2013) identified a salvage pathway in Gram-negative bacteria that bypasses de novo biosynthesis of UDP-MurNAc, offering a rationale for intrinsic fosfomycin resistance (Gisin et al., 2013).
Role in Antibiotic Resistance
Renner-Schneck et al. (2015) provided the first structural analysis of MurNAc-α1-P uridylyltransferase MurU, part of a cell wall recycling pathway in Gram-negative bacteria that contributes to high intrinsic resistance to fosfomycin (Renner-Schneck et al., 2015).
Exploration of Ligase Function
Kurokawa et al. (2007) explored Staphylococcus aureus MurC's role in L-alanine recognition, crucial for peptidoglycan biosynthesis. They characterized a temperature-sensitive mutation, providing experimental evidence supporting MurC's structural information (Kurokawa et al., 2007).
Impact on Peptidoglycan Production
Hervé et al. (2007) studied Escherichia coli's UDP-N-acetylmuramate:l-Alanyl-γ-d-Glutamyl-meso-Diaminopimelate ligase, highlighting its recycling role in peptidoglycan biosynthesis (Hervé, Boniface, Gobec, Blanot, & Mengin-Lecreulx, 2007).
Drug Development
Frlan et al. (2008) designed N-benzylidenesulfonohydrazide compounds as inhibitors of UDP-N-acetylmuramic acid:L-alanine ligase, essential in bacterial cell-wall biosynthesis, indicating potential as antibacterial agents (Frlan et al., 2008).
properties
Product Name |
UDP-N-acetylmuramic acid |
---|---|
Molecular Formula |
C20H28N3O19P2-3 |
Molecular Weight |
676.4 g/mol |
IUPAC Name |
(2R)-2-[(2R,3R,4R,5S,6R)-3-acetamido-2-[[[(2R,3S,4R,5R)-5-(2,4-dioxopyrimidin-1-yl)-3,4-dihydroxyoxolan-2-yl]methoxy-oxidophosphoryl]oxy-oxidophosphoryl]oxy-5-hydroxy-6-(hydroxymethyl)oxan-4-yl]oxypropanoate |
InChI |
InChI=1S/C20H31N3O19P2/c1-7(18(30)31)38-16-12(21-8(2)25)19(40-9(5-24)14(16)28)41-44(35,36)42-43(33,34)37-6-10-13(27)15(29)17(39-10)23-4-3-11(26)22-20(23)32/h3-4,7,9-10,12-17,19,24,27-29H,5-6H2,1-2H3,(H,21,25)(H,30,31)(H,33,34)(H,35,36)(H,22,26,32)/p-3/t7-,9-,10-,12-,13-,14-,15-,16-,17-,19-/m1/s1 |
InChI Key |
NQBRVZNDBBMBLJ-MQTLHLSBSA-K |
Isomeric SMILES |
C[C@H](C(=O)[O-])O[C@@H]1[C@H]([C@H](O[C@@H]([C@H]1O)CO)OP(=O)([O-])OP(=O)([O-])OC[C@@H]2[C@H]([C@H]([C@@H](O2)N3C=CC(=O)NC3=O)O)O)NC(=O)C |
SMILES |
CC(C(=O)[O-])OC1C(C(OC(C1O)CO)OP(=O)([O-])OP(=O)([O-])OCC2C(C(C(O2)N3C=CC(=O)NC3=O)O)O)NC(=O)C |
Canonical SMILES |
CC(C(=O)[O-])OC1C(C(OC(C1O)CO)OP(=O)([O-])OP(=O)([O-])OCC2C(C(C(O2)N3C=CC(=O)NC3=O)O)O)NC(=O)C |
synonyms |
Acetylmuramic Acid, UDP Acid, UDP Acetylmuramic UDP Acetylmuramic Acid Uridine Diphosphate N Acetylmuramic Acid Uridine Diphosphate N-Acetylmuramic Acid |
Origin of Product |
United States |
Retrosynthesis Analysis
AI-Powered Synthesis Planning: Our tool employs the Template_relevance Pistachio, Template_relevance Bkms_metabolic, Template_relevance Pistachio_ringbreaker, Template_relevance Reaxys, Template_relevance Reaxys_biocatalysis model, leveraging a vast database of chemical reactions to predict feasible synthetic routes.
One-Step Synthesis Focus: Specifically designed for one-step synthesis, it provides concise and direct routes for your target compounds, streamlining the synthesis process.
Accurate Predictions: Utilizing the extensive PISTACHIO, BKMS_METABOLIC, PISTACHIO_RINGBREAKER, REAXYS, REAXYS_BIOCATALYSIS database, our tool offers high-accuracy predictions, reflecting the latest in chemical research and data.
Strategy Settings
Precursor scoring | Relevance Heuristic |
---|---|
Min. plausibility | 0.01 |
Model | Template_relevance |
Template Set | Pistachio/Bkms_metabolic/Pistachio_ringbreaker/Reaxys/Reaxys_biocatalysis |
Top-N result to add to graph | 6 |
Feasible Synthetic Routes
Disclaimer and Information on In-Vitro Research Products
Please be aware that all articles and product information presented on BenchChem are intended solely for informational purposes. The products available for purchase on BenchChem are specifically designed for in-vitro studies, which are conducted outside of living organisms. In-vitro studies, derived from the Latin term "in glass," involve experiments performed in controlled laboratory settings using cells or tissues. It is important to note that these products are not categorized as medicines or drugs, and they have not received approval from the FDA for the prevention, treatment, or cure of any medical condition, ailment, or disease. We must emphasize that any form of bodily introduction of these products into humans or animals is strictly prohibited by law. It is essential to adhere to these guidelines to ensure compliance with legal and ethical standards in research and experimentation.