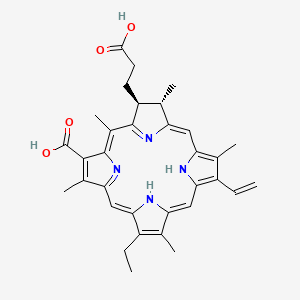
Chlorin E4
Overview
Description
Chlorin E4 is an organic compound that belongs to the family of chlorins, which are macrocyclic compounds with a structure similar to porphyrins . It is commonly used to improve photodynamic therapy for cancer and other diseases . Chlorin E4 has multiple applications in medical research, including as a photosensitizer for localized tumor destruction .
Synthesis Analysis
Chlorin E4 can be synthesized through the derivatization of porphyrins. This approach involves hydrogenation, cycloaddition, annulation, and various “breaking and mending” techniques. Another method involves the esterification of chlorin e6 with amino alcohols, leading to cationic water-soluble esters.Molecular Structure Analysis
The molecular structure of Chlorin E4, characterized by its dihydroporphyrin macrocycle, includes one pyrroline ring and three pyrrole rings. This structure is central to its function and similar to the core chromophore of chlorophyll.Chemical Reactions Analysis
Chlorin E4 undergoes various chemical reactions, reflecting its reactivity and potential for modification. For instance, the synthesis of amide derivatives of chlorin e6 showcases the molecule’s versatility in forming secondary and tertiary amides under mild conditions. These reactions demonstrate the compound’s ability to engage in addition, substitution, and electron transfer processes, significantly influencing its chemical behavior and utility.Scientific Research Applications
Photodynamic Therapy (PDT) for Cancer Treatment
Chlorin E4 has been utilized in PDT, where it’s activated by light to produce reactive oxygen species that can kill cancer cells. The sodium salt of Ce4 has been patented for use in PDT for various cancers, including ovarian cancer . Its ability to absorb light in the 600–700 nm range, where tissue penetration is deeper, makes it an effective agent for targeting tumor cells .
Intracellular Localization and Metabolic Profiling
High-resolution magic angle spinning NMR spectroscopy has been employed to study the metabolic profile of cultured HeLa cells treated with Ce4. This research has shown that Ce4 can localize within cell membranes, affecting the cellular phospholipid choline resonance . Such studies are crucial for understanding the intracellular fate of photosensitizers and their impact on cell metabolism .
Carrier Systems for Photosensitizers
Ce4’s effectiveness can be influenced by its carrier system. Studies have compared Ce4 alone to Ce4 encapsulated in carriers like polyvinylpyrrolidone (PVP) and the micelle-forming polyethylene glycol-polypropylene glycol triblock copolymer Kolliphor P188 (KP). These carriers can affect the intracellular uptake and distribution of Ce4, as well as its metabolic impact on cells .
Photophysical Property Maintenance
Maintaining the photophysical properties of Ce4 in aqueous solutions is vital for its application in PDT. Research has focused on preventing aggregation and ensuring that Ce4 retains its properties when used in medical applications .
Metabolic Response to Photosensitizers
The metabolic response of cells to Ce4 has been studied using multivariate statistical analyses of NMR spectra. This includes observing alterations in metabolites involved in the tricarboxylic acid cycle and phosphatidylcholine metabolism, which are significant for understanding the therapeutic effects and side effects of Ce4 .
Nanoparticle Effects on Photosensitizers
The interaction between nanoparticles and photosensitizers like Ce4 is a growing field of study. Research indicates that nanoparticles can affect the dark toxicity, side effects, and in vivo tolerability of photosensitizers. Understanding these interactions is key for developing safe and effective PDT treatments .
Mechanism of Action
Target of Action
Chlorin E4, an organic compound belonging to the family of chlorins, is structurally similar to porphyrins . It primarily targets mammalian phase 2 proteins . These proteins play a crucial role in protecting cells against oxidants and electrophiles .
Mode of Action
Chlorin E4 interacts with its targets by inducing mammalian phase 2 proteins that protect cells against oxidants and electrophiles . This induction depends on the ability of Chlorin E4 or its metabolites to react with thiol groups . This property is shared with all other classes of phase 2 inducers .
Biochemical Pathways
Chlorin E4 affects the tricarboxylic acid cycle and the phosphatidylcholine metabolism . It induces significant alterations in these metabolic pathways, which are concentration-dependent .
Pharmacokinetics
Studies have shown that chlorin e4 exhibits membrane localization in cells, as evidenced by its concentration-dependent chemical shift perturbation of the cellular phospholipid choline resonance .
Result of Action
The primary result of Chlorin E4’s action is the induction of mammalian phase 2 proteins, which protect cells against harmful oxidants and electrophiles . This results in enhanced cellular protection and potentially contributes to the prevention of diseases like cancer .
Action Environment
The action, efficacy, and stability of Chlorin E4 can be influenced by various environmental factors. For instance, the presence of carrier systems like polyvinylpyrrolidone (PVP) or the micelle-forming polyethylene glycol (PEG)-polypropylene glycol triblock copolymer Kolliphor P188 (KP) can affect the intracellular fate of Chlorin E4 . These carriers can modulate the concentration-dependent metabolic response upon exposure to Chlorin E4 .
properties
IUPAC Name |
(17S,18S)-18-(2-carboxyethyl)-12-ethenyl-7-ethyl-3,8,13,17,20-pentamethyl-17,18,22,23-tetrahydroporphyrin-2-carboxylic acid | |
---|---|---|
Source | PubChem | |
URL | https://pubchem.ncbi.nlm.nih.gov | |
Description | Data deposited in or computed by PubChem | |
InChI |
InChI=1S/C33H36N4O4/c1-8-20-15(3)23-12-25-17(5)22(10-11-29(38)39)31(36-25)19(7)32-30(33(40)41)18(6)26(37-32)14-28-21(9-2)16(4)24(35-28)13-27(20)34-23/h8,12-14,17,22,34-35H,1,9-11H2,2-7H3,(H,38,39)(H,40,41)/t17-,22-/m0/s1 | |
Source | PubChem | |
URL | https://pubchem.ncbi.nlm.nih.gov | |
Description | Data deposited in or computed by PubChem | |
InChI Key |
AKQWLZLQQVVKCI-JTSKRJEESA-N | |
Source | PubChem | |
URL | https://pubchem.ncbi.nlm.nih.gov | |
Description | Data deposited in or computed by PubChem | |
Canonical SMILES |
CCC1=C(C2=CC3=C(C(=C(N3)C=C4C(C(C(=N4)C(=C5C(=C(C(=N5)C=C1N2)C)C(=O)O)C)CCC(=O)O)C)C)C=C)C | |
Source | PubChem | |
URL | https://pubchem.ncbi.nlm.nih.gov | |
Description | Data deposited in or computed by PubChem | |
Isomeric SMILES |
CCC1=C(C2=CC3=C(C(=C(N3)C=C4[C@H]([C@@H](C(=N4)C(=C5C(=C(C(=N5)C=C1N2)C)C(=O)O)C)CCC(=O)O)C)C)C=C)C | |
Source | PubChem | |
URL | https://pubchem.ncbi.nlm.nih.gov | |
Description | Data deposited in or computed by PubChem | |
Molecular Formula |
C33H36N4O4 | |
Source | PubChem | |
URL | https://pubchem.ncbi.nlm.nih.gov | |
Description | Data deposited in or computed by PubChem | |
Molecular Weight |
552.7 g/mol | |
Source | PubChem | |
URL | https://pubchem.ncbi.nlm.nih.gov | |
Description | Data deposited in or computed by PubChem | |
Product Name |
Chlorin E4 |
Retrosynthesis Analysis
AI-Powered Synthesis Planning: Our tool employs the Template_relevance Pistachio, Template_relevance Bkms_metabolic, Template_relevance Pistachio_ringbreaker, Template_relevance Reaxys, Template_relevance Reaxys_biocatalysis model, leveraging a vast database of chemical reactions to predict feasible synthetic routes.
One-Step Synthesis Focus: Specifically designed for one-step synthesis, it provides concise and direct routes for your target compounds, streamlining the synthesis process.
Accurate Predictions: Utilizing the extensive PISTACHIO, BKMS_METABOLIC, PISTACHIO_RINGBREAKER, REAXYS, REAXYS_BIOCATALYSIS database, our tool offers high-accuracy predictions, reflecting the latest in chemical research and data.
Strategy Settings
Precursor scoring | Relevance Heuristic |
---|---|
Min. plausibility | 0.01 |
Model | Template_relevance |
Template Set | Pistachio/Bkms_metabolic/Pistachio_ringbreaker/Reaxys/Reaxys_biocatalysis |
Top-N result to add to graph | 6 |
Feasible Synthetic Routes
Disclaimer and Information on In-Vitro Research Products
Please be aware that all articles and product information presented on BenchChem are intended solely for informational purposes. The products available for purchase on BenchChem are specifically designed for in-vitro studies, which are conducted outside of living organisms. In-vitro studies, derived from the Latin term "in glass," involve experiments performed in controlled laboratory settings using cells or tissues. It is important to note that these products are not categorized as medicines or drugs, and they have not received approval from the FDA for the prevention, treatment, or cure of any medical condition, ailment, or disease. We must emphasize that any form of bodily introduction of these products into humans or animals is strictly prohibited by law. It is essential to adhere to these guidelines to ensure compliance with legal and ethical standards in research and experimentation.