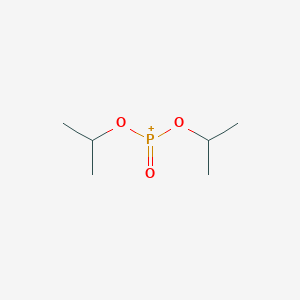
Diisopropyl phosphonate
Overview
Description
The Diisopropylphosphono Group is an organic compound belonging to the class of phosphonic acid diesters. These compounds contain a diester derivative of phosphonic acid, with the general structure ROP(=O)OR’ (where R and R’ are organic groups). The chemical formula for the Diisopropylphosphono Group is C6H15O3P .
Mechanism of Action
Target of Action
The primary targets of Diisopropyl phosphonate are enzymes such as Parathion hydrolase . These enzymes are found in organisms like Brevundimonas diminuta and Flavobacterium sp . The compound interacts with these enzymes, affecting their function and activity.
Mode of Action
This compound interacts with its targets by catalyzing the hydrolysis of certain substrates . For instance, it catalyzes the hydrolysis of the insecticide paraoxon at a rate approaching the diffusion limit . This suggests that the compound has evolved to optimally utilize this synthetic substrate.
Biochemical Pathways
This compound affects various biochemical pathways. As a phosphonate, it mimics the phosphates and carboxylates of biological molecules, potentially inhibiting metabolic enzymes . This can lead to changes in the downstream effects of these pathways, impacting various biological processes.
Pharmacokinetics
It’s known that once inside the body, related compounds like diisopropyl methylphosphonate are rapidly converted to other forms and cleared from the blood .
Result of Action
The molecular and cellular effects of this compound’s action depend on its interaction with its targets and the specific biochemical pathways it affects. For example, its ability to catalyze the hydrolysis of certain substrates can lead to changes in the activity of certain enzymes, potentially affecting various biological processes .
Action Environment
Environmental factors can influence the action, efficacy, and stability of this compound. For instance, it’s known that most of the compound enters groundwater or surface water when it enters the environment . . These environmental factors can influence how the compound interacts with its targets and affects biochemical pathways.
Biochemical Analysis
Biochemical Properties
Diisopropyl phosphonate plays a significant role in biochemical reactions . It often inhibits enzymes utilizing various phosphates as substrates . The compound can be prepared from its esters, phosphinates and phosphonates, respectively, by hydrolysis or dealkylation .
Cellular Effects
It is known that phosphonates, a group to which this compound belongs, can have significant effects on cells . For instance, they can inhibit enzymes and affect various cellular processes .
Molecular Mechanism
The molecular mechanism of this compound is complex and involves several biochemical pathways . It is known to inhibit enzymes utilizing various phosphates as substrates
Temporal Effects in Laboratory Settings
It is known that the hydrolysis of phosphonate esters, a group to which this compound belongs, can take place both under acidic and basic conditions .
Metabolic Pathways
This compound is involved in several metabolic pathways . It is known to inhibit enzymes utilizing various phosphates as substrates
Transport and Distribution
It is known that phosphonates, a group to which this compound belongs, can be transported and distributed within cells and tissues .
Subcellular Localization
It is known that phosphonates, a group to which this compound belongs, can be localized in various subcellular compartments .
Preparation Methods
The synthesis of the Diisopropylphosphono Group typically involves the esterification of phosphonic acid with isopropanol. This reaction can be catalyzed by acids such as hydrochloric acid or sulfuric acid. The reaction conditions often require heating to facilitate the esterification process. Industrial production methods may involve continuous flow reactors to optimize yield and efficiency .
Chemical Reactions Analysis
The Diisopropylphosphono Group undergoes various chemical reactions, including:
Oxidation: It can be oxidized to form phosphonic acid derivatives.
Reduction: Reduction reactions can convert it into phosphine oxides.
Substitution: It can undergo nucleophilic substitution reactions where the isopropyl groups are replaced by other nucleophiles.
Common reagents used in these reactions include oxidizing agents like hydrogen peroxide, reducing agents such as lithium aluminum hydride, and nucleophiles like amines or alcohols. The major products formed from these reactions depend on the specific reagents and conditions used .
Scientific Research Applications
The Diisopropylphosphono Group has several applications in scientific research:
Chemistry: It is used as a reagent in organic synthesis, particularly in the formation of phosphonate esters.
Biology: It serves as a probe in enzymatic studies to understand the role of phosphonate esters in biological systems.
Medicine: It is investigated for its potential use in drug development, particularly in the design of enzyme inhibitors.
Comparison with Similar Compounds
The Diisopropylphosphono Group can be compared with other phosphonic acid diesters, such as:
- Dimethylphosphono Group
- Diethylphosphono Group
- Diphenylphosphono Group
What sets the Diisopropylphosphono Group apart is its unique combination of steric and electronic properties conferred by the isopropyl groups. These properties influence its reactivity and stability, making it suitable for specific applications where other phosphonic acid diesters may not be as effective .
Properties
IUPAC Name |
oxo-di(propan-2-yloxy)phosphanium | |
---|---|---|
Source | PubChem | |
URL | https://pubchem.ncbi.nlm.nih.gov | |
Description | Data deposited in or computed by PubChem | |
InChI |
InChI=1S/C6H14O3P/c1-5(2)8-10(7)9-6(3)4/h5-6H,1-4H3/q+1 | |
Source | PubChem | |
URL | https://pubchem.ncbi.nlm.nih.gov | |
Description | Data deposited in or computed by PubChem | |
InChI Key |
BJLZAAWLLPMZQR-UHFFFAOYSA-N | |
Source | PubChem | |
URL | https://pubchem.ncbi.nlm.nih.gov | |
Description | Data deposited in or computed by PubChem | |
Canonical SMILES |
CC(C)O[P+](=O)OC(C)C | |
Source | PubChem | |
URL | https://pubchem.ncbi.nlm.nih.gov | |
Description | Data deposited in or computed by PubChem | |
Molecular Formula |
C6H14O3P+ | |
Source | PubChem | |
URL | https://pubchem.ncbi.nlm.nih.gov | |
Description | Data deposited in or computed by PubChem | |
DSSTOX Substance ID |
DTXSID7061988 | |
Record name | Diisopropylphosphite | |
Source | EPA DSSTox | |
URL | https://comptox.epa.gov/dashboard/DTXSID7061988 | |
Description | DSSTox provides a high quality public chemistry resource for supporting improved predictive toxicology. | |
Molecular Weight |
165.15 g/mol | |
Source | PubChem | |
URL | https://pubchem.ncbi.nlm.nih.gov | |
Description | Data deposited in or computed by PubChem | |
CAS No. |
1809-20-7 | |
Record name | O,O-Diisopropyl phosphite | |
Source | ChemIDplus | |
URL | https://pubchem.ncbi.nlm.nih.gov/substance/?source=chemidplus&sourceid=0001809207 | |
Description | ChemIDplus is a free, web search system that provides access to the structure and nomenclature authority files used for the identification of chemical substances cited in National Library of Medicine (NLM) databases, including the TOXNET system. | |
Record name | Diisopropyl phosphonate | |
Source | DTP/NCI | |
URL | https://dtp.cancer.gov/dtpstandard/servlet/dwindex?searchtype=NSC&outputformat=html&searchlist=82344 | |
Description | The NCI Development Therapeutics Program (DTP) provides services and resources to the academic and private-sector research communities worldwide to facilitate the discovery and development of new cancer therapeutic agents. | |
Explanation | Unless otherwise indicated, all text within NCI products is free of copyright and may be reused without our permission. Credit the National Cancer Institute as the source. | |
Record name | Phosphonic acid, bis(1-methylethyl) ester | |
Source | EPA Chemicals under the TSCA | |
URL | https://www.epa.gov/chemicals-under-tsca | |
Description | EPA Chemicals under the Toxic Substances Control Act (TSCA) collection contains information on chemicals and their regulations under TSCA, including non-confidential content from the TSCA Chemical Substance Inventory and Chemical Data Reporting. | |
Record name | Diisopropylphosphite | |
Source | EPA DSSTox | |
URL | https://comptox.epa.gov/dashboard/DTXSID7061988 | |
Description | DSSTox provides a high quality public chemistry resource for supporting improved predictive toxicology. | |
Record name | Diisopropyl phosphonate | |
Source | European Chemicals Agency (ECHA) | |
URL | https://echa.europa.eu/substance-information/-/substanceinfo/100.015.743 | |
Description | The European Chemicals Agency (ECHA) is an agency of the European Union which is the driving force among regulatory authorities in implementing the EU's groundbreaking chemicals legislation for the benefit of human health and the environment as well as for innovation and competitiveness. | |
Explanation | Use of the information, documents and data from the ECHA website is subject to the terms and conditions of this Legal Notice, and subject to other binding limitations provided for under applicable law, the information, documents and data made available on the ECHA website may be reproduced, distributed and/or used, totally or in part, for non-commercial purposes provided that ECHA is acknowledged as the source: "Source: European Chemicals Agency, http://echa.europa.eu/". Such acknowledgement must be included in each copy of the material. ECHA permits and encourages organisations and individuals to create links to the ECHA website under the following cumulative conditions: Links can only be made to webpages that provide a link to the Legal Notice page. | |
Record name | DIISOPROPYL PHOSPHONATE | |
Source | FDA Global Substance Registration System (GSRS) | |
URL | https://gsrs.ncats.nih.gov/ginas/app/beta/substances/676G4RQ6ND | |
Description | The FDA Global Substance Registration System (GSRS) enables the efficient and accurate exchange of information on what substances are in regulated products. Instead of relying on names, which vary across regulatory domains, countries, and regions, the GSRS knowledge base makes it possible for substances to be defined by standardized, scientific descriptions. | |
Explanation | Unless otherwise noted, the contents of the FDA website (www.fda.gov), both text and graphics, are not copyrighted. They are in the public domain and may be republished, reprinted and otherwise used freely by anyone without the need to obtain permission from FDA. Credit to the U.S. Food and Drug Administration as the source is appreciated but not required. | |
Retrosynthesis Analysis
AI-Powered Synthesis Planning: Our tool employs the Template_relevance Pistachio, Template_relevance Bkms_metabolic, Template_relevance Pistachio_ringbreaker, Template_relevance Reaxys, Template_relevance Reaxys_biocatalysis model, leveraging a vast database of chemical reactions to predict feasible synthetic routes.
One-Step Synthesis Focus: Specifically designed for one-step synthesis, it provides concise and direct routes for your target compounds, streamlining the synthesis process.
Accurate Predictions: Utilizing the extensive PISTACHIO, BKMS_METABOLIC, PISTACHIO_RINGBREAKER, REAXYS, REAXYS_BIOCATALYSIS database, our tool offers high-accuracy predictions, reflecting the latest in chemical research and data.
Strategy Settings
Precursor scoring | Relevance Heuristic |
---|---|
Min. plausibility | 0.01 |
Model | Template_relevance |
Template Set | Pistachio/Bkms_metabolic/Pistachio_ringbreaker/Reaxys/Reaxys_biocatalysis |
Top-N result to add to graph | 6 |
Feasible Synthetic Routes
Disclaimer and Information on In-Vitro Research Products
Please be aware that all articles and product information presented on BenchChem are intended solely for informational purposes. The products available for purchase on BenchChem are specifically designed for in-vitro studies, which are conducted outside of living organisms. In-vitro studies, derived from the Latin term "in glass," involve experiments performed in controlled laboratory settings using cells or tissues. It is important to note that these products are not categorized as medicines or drugs, and they have not received approval from the FDA for the prevention, treatment, or cure of any medical condition, ailment, or disease. We must emphasize that any form of bodily introduction of these products into humans or animals is strictly prohibited by law. It is essential to adhere to these guidelines to ensure compliance with legal and ethical standards in research and experimentation.