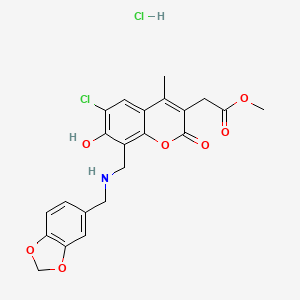
C22H21Cl2NO7
- Click on QUICK INQUIRY to receive a quote from our team of experts.
- With the quality product at a COMPETITIVE price, you can focus more on your research.
Overview
Description
The compound with the molecular formula C22H21Cl2NO7 Loratadine . It is a second-generation antihistamine used to treat allergies. Unlike first-generation antihistamines, Loratadine does not readily cross the blood-brain barrier, which minimizes its sedative effects .
Preparation Methods
Synthetic Routes and Reaction Conditions
Loratadine can be synthesized through a multi-step process. One common method involves the reaction of 4-(8-chloro-5,6-dihydro-11H-benzo[5,6]cyclohepta[1,2-b]pyridin-11-ylidene)-1-piperidinecarboxylic acid ethyl ester with ethyl chloroformate in the presence of a base such as triethylamine . The reaction is typically carried out in a solvent like toluene at a temperature of around 0-5°C .
Industrial Production Methods
In industrial settings, Loratadine is produced using similar synthetic routes but on a larger scale. The process involves stringent quality control measures to ensure the purity and efficacy of the final product. The reaction conditions are optimized to maximize yield and minimize impurities .
Chemical Reactions Analysis
Types of Reactions
Loratadine undergoes several types of chemical reactions, including:
Oxidation: Loratadine can be oxidized to form its active metabolite, .
Hydrolysis: The ester group in Loratadine can be hydrolyzed under acidic or basic conditions to yield the corresponding carboxylic acid.
Substitution: Loratadine can undergo nucleophilic substitution reactions, particularly at the chlorine atom.
Common Reagents and Conditions
Oxidation: Common oxidizing agents include and .
Hydrolysis: Acidic hydrolysis can be carried out using , while basic hydrolysis can be performed using .
Substitution: Nucleophilic substitution reactions often use reagents like sodium azide or potassium cyanide .
Major Products
Desloratadine: Formed through oxidation.
Carboxylic Acid Derivatives: Formed through hydrolysis.
Substituted Derivatives: Formed through nucleophilic substitution.
Scientific Research Applications
Loratadine has a wide range of applications in scientific research:
Chemistry: Used as a model compound to study the pharmacokinetics and metabolism of antihistamines.
Biology: Employed in studies investigating the role of histamine in allergic reactions and immune responses.
Medicine: Widely used in clinical trials to evaluate its efficacy and safety in treating various allergic conditions.
Industry: Utilized in the formulation of over-the-counter allergy medications.
Mechanism of Action
Loratadine exerts its effects by selectively inhibiting peripheral histamine H1 receptors . This prevents histamine from binding to its receptors, thereby reducing the symptoms of allergic reactions such as itching, swelling, and redness. Loratadine does not significantly cross the blood-brain barrier, which minimizes central nervous system side effects .
Comparison with Similar Compounds
Similar Compounds
Cetirizine: Another second-generation antihistamine with similar efficacy but a higher likelihood of causing drowsiness.
Fexofenadine: Known for its non-sedative properties and longer duration of action.
Desloratadine: The active metabolite of Loratadine, offering similar benefits with potentially fewer side effects.
Uniqueness
Loratadine is unique in its balance of efficacy and minimal sedative effects. Its ability to selectively target peripheral histamine H1 receptors while avoiding significant central nervous system penetration makes it a preferred choice for many patients .
Biological Activity
C22H21Cl2NO7 is a complex organic compound that has garnered attention for its potential biological activities, particularly in the fields of oncology and microbiology. This article delves into the compound's biological properties, mechanisms of action, and relevant case studies, supported by data tables and research findings.
Chemical Structure and Properties
This compound belongs to a class of compounds known as prodigiosenes, which are characterized by their unique pyrrolylpyrromethene skeleton. The presence of chlorine atoms and a nitrogen-containing moiety contributes to its biological activity. The compound's structure can be represented as follows:
The biological activity of this compound is attributed to several mechanisms:
- Cytotoxicity : The compound exhibits significant cytotoxic effects against various cancer cell lines. Studies have shown that it induces apoptosis through multiple pathways, including DNA cleavage mediated by Cu(II) complexes .
- Antimicrobial Activity : this compound demonstrates antimicrobial properties, particularly against Staphylococcus aureus strains. This activity is linked to its ability to disrupt bacterial cell membranes and inhibit vital cellular functions .
- Topoisomerase Inhibition : The compound acts as a dual topoisomerase inhibitor, which is crucial for DNA replication and transcription. This mechanism further enhances its potential as an anticancer agent .
Biological Activity Data
The following table summarizes the biological activities observed for this compound in various studies:
Case Studies
Several case studies have highlighted the efficacy of this compound in clinical and experimental settings:
- Cancer Treatment : A study investigated the cytotoxic effects of this compound on various tumor cell lines. Results indicated a strong correlation between the concentration of the compound and cell viability, with significant apoptosis observed at higher concentrations .
- Infection Control : Another case study focused on the antimicrobial properties of this compound against resistant bacterial strains. The compound demonstrated effective inhibition of bacterial growth, suggesting its potential use in treating infections caused by multidrug-resistant organisms .
- Photodynamic Therapy (PDT) : Research has explored the use of this compound in photodynamic therapy, where it showed enhanced anticancer activity when activated by light. This method leverages the compound's ability to generate reactive oxygen species upon light exposure, leading to targeted cell death .
Research Findings
Recent findings emphasize the need for further exploration into the structure-activity relationship (SAR) of this compound:
- The hydrophobic substituents significantly influence its biological activity, particularly in terms of cytotoxicity and antimicrobial effects .
- Investigations into Cu(II) complexation reveal that these interactions are crucial for enhancing the compound's efficacy against cancer cells and pathogens .
- Future studies should focus on optimizing the substitution patterns on the prodigiosene scaffold to improve selectivity and potency against specific targets.
Properties
Molecular Formula |
C22H21Cl2NO7 |
---|---|
Molecular Weight |
482.3 g/mol |
IUPAC Name |
methyl 2-[8-[(1,3-benzodioxol-5-ylmethylamino)methyl]-6-chloro-7-hydroxy-4-methyl-2-oxochromen-3-yl]acetate;hydrochloride |
InChI |
InChI=1S/C22H20ClNO7.ClH/c1-11-13-6-16(23)20(26)15(21(13)31-22(27)14(11)7-19(25)28-2)9-24-8-12-3-4-17-18(5-12)30-10-29-17;/h3-6,24,26H,7-10H2,1-2H3;1H |
InChI Key |
HSAFQXYXPFDPKQ-UHFFFAOYSA-N |
Canonical SMILES |
CC1=C(C(=O)OC2=C(C(=C(C=C12)Cl)O)CNCC3=CC4=C(C=C3)OCO4)CC(=O)OC.Cl |
Origin of Product |
United States |
Disclaimer and Information on In-Vitro Research Products
Please be aware that all articles and product information presented on BenchChem are intended solely for informational purposes. The products available for purchase on BenchChem are specifically designed for in-vitro studies, which are conducted outside of living organisms. In-vitro studies, derived from the Latin term "in glass," involve experiments performed in controlled laboratory settings using cells or tissues. It is important to note that these products are not categorized as medicines or drugs, and they have not received approval from the FDA for the prevention, treatment, or cure of any medical condition, ailment, or disease. We must emphasize that any form of bodily introduction of these products into humans or animals is strictly prohibited by law. It is essential to adhere to these guidelines to ensure compliance with legal and ethical standards in research and experimentation.