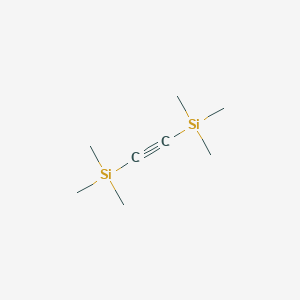
Bis(trimethylsilyl)acetylene
Overview
Description
Bis(trimethylsilyl)acetylene (BTMSA, C₈H₁₈Si₂) is a silicon-substituted alkyne characterized by two trimethylsilyl (TMS) groups attached to an acetylene core. Its unique steric and electronic properties, derived from the bulky TMS substituents, make it a versatile reagent in organic synthesis, organometallic chemistry, and materials science. BTMSA is commercially available, cost-effective, and widely used in reactions such as silylation, coupling, and as a precursor for carbon-rich materials .
Preparation Methods
Traditional Lithiation-Silylation Method
The most widely documented synthesis of BTMSA involves sequential lithiation of acetylene followed by silylation. As detailed in foundational studies, acetylene gas is first treated with n-butyllithium in an anhydrous tetrahydrofuran (THF) medium at low temperatures (-78°C) to generate lithium acetylide (LiC≡CLi) . This intermediate reacts exothermically with trimethylsilyl chloride (Me₃SiCl), yielding BTMSA and lithium chloride as a byproduct .
Reaction Mechanism and Conditions
The lithiation step proceeds via deprotonation of acetylene by n-BuLi, forming a dilithium acetylide complex. Subsequent quench with Me₃SiCl introduces trimethylsilyl groups at both terminal positions. The reaction demands strict anhydrous conditions and inert gas purging to prevent hydrolysis of intermediates . Purification typically involves fractional distillation under reduced pressure, achieving yields exceeding 85% .
Diethylzinc-Mediated Silylation Approach
A modified protocol employing diethylzinc (Et₂Zn) as a base was developed to circumvent the hazards of organolithium reagents. Huang et al. (2019) demonstrated that BTMSA could be synthesized by reacting acetylene with chlorotrimethylsilane in hexane/acetonitrile at 80°C for 20 hours under Schlenk conditions .
Optimized Procedure
In a representative procedure, acetylene (2 mmol) and chlorotrimethylsilane (2 mmol) are combined with Et₂Zn (1 mL of 1 M solution in hexane) in acetonitrile. The mixture is stirred at 80°C under nitrogen, followed by solvent evaporation and silica gel chromatography (hexane/ethyl acetate). This method achieves a 91% yield, significantly higher than traditional routes .
Advantages Over Lithiation
Et₂Zn’s milder reactivity reduces side reactions, enabling higher selectivity. The use of acetonitrile as a polar aprotic solvent enhances reaction rates by stabilizing ionic intermediates . However, Schlenk techniques and rigorous exclusion of moisture remain essential, limiting its adoption in resource-constrained settings.
Calcium Carbide and N-(Trimethylsilyl)imidazole Reaction
A groundbreaking 2020 study introduced a novel pathway utilizing calcium carbide (CaC₂) and N-(trimethylsilyl)imidazole (TMSIm) in dichloromethane, catalyzed by copper(I) bromide (CuBr) . This method bypasses gaseous acetylene entirely, enhancing operational safety.
Reaction Dynamics
CaC₂ reacts directly with TMSIm at 100°C, with CuBr facilitating silyl group transfer. Control experiments confirmed the absence of free acetylene intermediates, suggesting a concerted mechanism where CaC₂’s lattice acetylide bonds undergo nucleophilic attack by TMSIm . The reaction completes within 24 hours, yielding 68.8% BTMSA after extraction with dichloromethane .
Industrial Prospects
This route eliminates gas-handling infrastructure and protonated solvents, reducing costs and environmental impact. However, the moderate yield and high catalyst loading (10 mol% CuBr) necessitate further optimization for commercial viability .
Comparative Analysis of Synthesis Methods
Chemical Reactions Analysis
Cycloaddition Reactions
BTMSA participates in [2+2], [3+2], and [4+2] cycloadditions due to its electron-rich alkyne moiety.
Diels-Alder Reactions
Substrate | Conditions | Product | Yield | Ref. |
---|---|---|---|---|
Acetophenone | KHMDS (3 mol%), BTMSA (2 eq.), 80°C | β-Silylated propargyl alcohol | 95% | |
Diarylacetylenes | Rh(OH)(cod)/bisphosphine, phenol | Anti-selective cross-adduct | 80% |
Key findings :
-
BTMSA reacts with ketones under base catalysis (e.g., KHMDS) to form silylated propargyl alcohols .
-
Rhodium-catalyzed anti-selective additions to diarylacetylenes occur via C–Si bond cleavage .
Coordination Chemistry
BTMSA serves as a ligand in low-valent metallocene complexes:
Group 4 Metallocene Complexation
Complex | Reaction | Application | Ref. |
---|---|---|---|
CpTi(η-btmsa) | CpTiCl + Mg + BTMSA → CpTi[(CSiMe)] + MgCl | Precursor to reactive Ti centers | |
CpZr(py)(btmsa) | CpZrCl reduction in presence of BTMSA | Coupling reactions |
Mechanistic Insight :
-
BTMSA dissociates to generate coordinatively unsaturated [CpM] fragments, enabling substrate activation (e.g., carbodiimide C–N bond cleavage) .
Cross-Coupling Reactions
BTMSA is utilized in Stille, Suzuki, and sila-Sonogashira couplings:
Stille Coupling Example
Protiodesilylation
Substrate | Conditions | Product | Yield | Ref. |
---|---|---|---|---|
MeSiC≡C–R | KCO/MeOH | HC≡C–R | >90% |
Thermal Behavior
BTMSA enhances carbon yield (15–20%) in cellulose-derived carbon fibers by stabilizing intermediates during pyrolysis .
Scientific Research Applications
Catalytic Applications
1.1. Metallocene Complexes
BTMSA is extensively used in the formation of metallocene complexes, particularly with group 4 metals. These complexes are crucial for various catalytic processes, including polymerization and hydrogenation reactions. The advantages of using BTMSA in these complexes include:
- Stability : BTMSA forms stable complexes that can be activated under mild conditions.
- Reactivity : These complexes exhibit high reactivity due to the electronic properties imparted by the silicon atoms in BTMSA.
For example, a study demonstrated that metallocene complexes with BTMSA could effectively catalyze the formation of reactive intermediates necessary for further synthetic transformations .
1.2. Base-Catalyzed Reactions
BTMSA has been utilized in base-catalyzed reactions with ketones to produce silyl-protected propargylic alcohols. This method shows high efficiency and selectivity, yielding products in 72-96% yield. The reaction mechanism involves the addition of BTMSA to carbonyl compounds, leading to the formation of valuable intermediates for further functionalization .
Material Science Applications
2.1. Carbon Fiber Precursors
Recent research highlights the role of BTMSA in enhancing the properties of carbon fiber precursors derived from cellulose. The incorporation of BTMSA into cellulose solutions significantly increases carbon yield during carbonization processes. Key findings include:
- Thermal Behavior : The introduction of BTMSA alters the thermal expansion coefficients of the resulting fibers, indicating improved thermal stability.
- Structural Features : The composite fibers exhibit distinct thermal properties compared to pure cellulose fibers, making them suitable for high-performance applications .
Synthetic Chemistry
3.1. Synthesis of Alkynols
BTMSA serves as a substrate for synthesizing terminal alkynols through chemoselective reactions with aldehydes and ketones. This one-step procedure simplifies the synthesis process while allowing for the recovery and reuse of BTMSA after the reaction .
Case Studies
Mechanism of Action
The mechanism of action of bis(trimethylsilyl)acetylene involves its role as a nucleophile and its ability to participate in cycloaddition reactions. It can form coordination complexes with central metal ions, such as titanium, to create reactive intermediates that facilitate various chemical transformations .
Comparison with Similar Compounds
Physical and Chemical Properties
The TMS groups in BTMSA significantly influence its physical properties, including melting point (21–24°C), boiling point (136–137°C), and density (0.752 g/mL) . These contrast with TMSA, which has a lower molecular weight (114.28 g/mol vs. 188.40 g/mol for BTMSA) and likely lower boiling point. TES acetylene, with triethylsilyl groups, exhibits higher hydrophobicity and thermal stability due to larger substituents but is less volatile .
Table 2: Physical Properties
Reactivity in Catalytic and Organometallic Reactions
Organometallic Complex Formation
BTMSA forms stable metallocene complexes with group 4 metals (e.g., Ti, Zr) due to strong η²-alkyne bonding. For example, [Cp₂Zr(py)(η²-BTMSA)] is air-stable and facilitates stoichiometric C–H activation and coupling reactions . In contrast, TMSA and TES acetylene form less stable complexes, as smaller substituents provide weaker metal-alkyne interactions .
Table 3: Reactivity Comparison
Biological Activity
Bis(trimethylsilyl)acetylene (BTMSA), with the chemical formula Me₃SiC≡CSiMe₃, is an organosilicon compound notable for its unique structural and chemical properties. This compound is primarily utilized in organic synthesis and catalysis, acting as a nucleophile in various reactions, including Friedel-Crafts acylations and alkylations. Its biological activity, while less extensively documented compared to its chemical applications, warrants exploration due to its potential implications in medicinal chemistry and material science.
BTMSA is synthesized through the reaction of acetylene with butyllithium followed by the addition of chlorotrimethylsilane. This process yields a crystalline solid that is soluble in organic solvents but not miscible with water . The compound's structure features two trimethylsilyl groups attached to an acetylene backbone, which influences its reactivity and interaction with biological systems.
Biological Activity Overview
While BTMSA's primary applications are in synthetic chemistry, its biological activity has been investigated in several contexts:
- Ligand Properties : BTMSA acts as a ligand in coordination chemistry, forming stable complexes with transition metals such as titanium and zirconium. These complexes have been explored for their catalytic properties, including hydrogenation reactions . The biological implications of these metal complexes are significant, as they may exhibit unique pharmacological activities.
- Toxicology : Limited data on the toxicity of BTMSA indicates that it should be handled with care, as organosilicon compounds can exhibit varying degrees of biological activity. The toxicity profile suggests potential irritative effects on the skin and eyes, but comprehensive studies are lacking .
Case Studies and Research Findings
Recent studies have highlighted the interactions of BTMSA-derived complexes with biological molecules:
- Catalytic Activity : Research has shown that BTMSA complexes can facilitate reactions involving small molecules such as carbon dioxide, leading to the formation of biologically relevant compounds. For instance, the interaction between BTMSA and permethyltitanocene has been studied for its potential to produce carbonate complexes that may have implications in carbon capture technologies .
- Reactivity with Biological Substrates : BTMSA has been utilized in synthetic pathways leading to biologically active molecules. For example, it has been employed in the total synthesis of natural products like epibatidine, a potent analgesic compound. This highlights its utility in drug discovery and development processes .
- Metallocene Complexes : The formation of metallocene complexes with BTMSA has opened avenues for exploring their catalytic roles in organic transformations that could lead to biologically active substances. These complexes exhibit unique reactivity patterns that could be harnessed for therapeutic applications .
Data Table: Summary of Biological Activity Studies
Q & A
Q. What are the critical safety considerations when handling BTMSA in laboratory settings?
BTMSA is a highly flammable liquid (flash point: 2–29°C) and a skin/eye irritant . Key protocols include:
- Storage : Maintain at 0–6°C in airtight containers to prevent decomposition or vapor accumulation .
- Handling : Use under inert gas (e.g., N₂/Ar) to avoid combustion risks. Employ spark-resistant tools and grounded equipment .
- PPE : Wear nitrile gloves, safety goggles, and flame-retardant lab coats. Use fume hoods for ventilation .
Q. How does BTMSA function as a ligand or reagent in organometallic synthesis?
BTMSA serves as a sterically protected alkyne in cross-coupling and cycloaddition reactions. For example:
- Cyclotrimerization : In Vollhardt’s benzocyclobutene synthesis, BTMSA acts as an exogenous alkyne in cobalt-catalyzed [2+2+2] cycloadditions, enabling regioselective C–C bond formation .
- Coordination Chemistry : It stabilizes low-valent metal centers (e.g., Ru, Ti) by donating electron density via the acetylene π-system, as seen in bimetallic ruthenium complexes .
Q. What methodologies optimize BTMSA’s reactivity in stereoselective C-glycosidation?
BTMSA’s silyl groups enhance nucleophilicity in glycosidation. Key steps include:
- Catalyst Choice : SnCl₄ mediates C-glycosidation between sugar acetates and BTMSA, achieving >90% yield by activating the glycosyl donor .
- Temperature Control : Reactions performed at –20°C to 0°C minimize side reactions (e.g., over-silylation) .
Q. How can electrochemical studies elucidate BTMSA’s role in redox-active complexes?
Cyclic voltammetry of BTMSA-derived ruthenium complexes reveals:
- Redox Peaks : A quasi-reversible Ru(II)/Ru(III) couple at +0.354 V and +0.874 V vs Ag/AgCl, indicating sequential one-electron oxidation .
- Ligand Effects : The silyl groups stabilize the metal center, reducing oxidation potentials compared to non-silylated analogs .
Q. What analytical challenges arise in characterizing BTMSA-derived products?
- Mass Spectrometry : Electron impact (EI-MS) induces fragmentation via Si–C bond cleavage, producing trimethylsilyl ions (m/z 73) and acetylene derivatives .
- NMR : ¹H and ¹³C NMR show distinct signals for silyl-protected alkynes (δ ~0.1 ppm for Si–CH₃; δ ~100–120 ppm for C≡C) .
Q. How do reaction conditions influence BTMSA’s stability in synthetic workflows?
While BTMSA is stable under inert atmospheres, prolonged heating (>100°C) or exposure to moisture can trigger decomposition. Mitigation strategies include:
- Solvent Selection : Use anhydrous THF or toluene to prevent hydrolysis .
- Catalyst Purity : Trace acids or bases (e.g., KOH) accelerate degradation; pre-dry catalysts at 120°C .
Q. What mechanistic insights explain BTMSA’s selectivity in alkyne coupling reactions?
In Cu(I)-catalyzed Glaser-type couplings:
- Silyl Protection : The trimethylsilyl groups block undesired homocoupling, enabling unsymmetrical diaryl acetylene synthesis .
- Kinetic Control : Lower temperatures (–40°C) favor cross-coupling over side reactions (e.g., oligomerization) .
Q. How does BTMSA compare to other silylated alkynes in synthetic applications?
- Steric Effects : BTMSA’s bulkier silyl groups provide better regioselectivity than (trimethylsilyl)acetylene in cycloadditions .
- Electronic Effects : Enhanced electron-donating capacity improves reactivity in low-valent metal complexes compared to non-silylated analogs .
Q. What contradictions exist in reported stability data for BTMSA?
While safety data sheets claim stability under "normal conditions" , storage recommendations (0–6°C) and decomposition risks at elevated temperatures suggest thermal instability. Researchers should validate stability via TGA/DSC before high-temperature applications .
Q. How is BTMSA utilized in advanced material synthesis (e.g., MOCVD)?
In metal-organic chemical vapor deposition (MOCVD):
Properties
IUPAC Name |
trimethyl(2-trimethylsilylethynyl)silane | |
---|---|---|
Source | PubChem | |
URL | https://pubchem.ncbi.nlm.nih.gov | |
Description | Data deposited in or computed by PubChem | |
InChI |
InChI=1S/C8H18Si2/c1-9(2,3)7-8-10(4,5)6/h1-6H3 | |
Source | PubChem | |
URL | https://pubchem.ncbi.nlm.nih.gov | |
Description | Data deposited in or computed by PubChem | |
InChI Key |
ZDWYFWIBTZJGOR-UHFFFAOYSA-N | |
Source | PubChem | |
URL | https://pubchem.ncbi.nlm.nih.gov | |
Description | Data deposited in or computed by PubChem | |
Canonical SMILES |
C[Si](C)(C)C#C[Si](C)(C)C | |
Source | PubChem | |
URL | https://pubchem.ncbi.nlm.nih.gov | |
Description | Data deposited in or computed by PubChem | |
Molecular Formula |
C8H18Si2 | |
Source | PubChem | |
URL | https://pubchem.ncbi.nlm.nih.gov | |
Description | Data deposited in or computed by PubChem | |
DSSTOX Substance ID |
DTXSID3065798 | |
Record name | Bis(trimethylsilyl)acetylene | |
Source | EPA DSSTox | |
URL | https://comptox.epa.gov/dashboard/DTXSID3065798 | |
Description | DSSTox provides a high quality public chemistry resource for supporting improved predictive toxicology. | |
Molecular Weight |
170.40 g/mol | |
Source | PubChem | |
URL | https://pubchem.ncbi.nlm.nih.gov | |
Description | Data deposited in or computed by PubChem | |
Physical Description |
Clear colorless liquid; mp = 21-24 deg C; [Sigma-Aldrich MSDS] | |
Record name | Bis(trimethylsilyl)acetylene | |
Source | Haz-Map, Information on Hazardous Chemicals and Occupational Diseases | |
URL | https://haz-map.com/Agents/20347 | |
Description | Haz-Map® is an occupational health database designed for health and safety professionals and for consumers seeking information about the adverse effects of workplace exposures to chemical and biological agents. | |
Explanation | Copyright (c) 2022 Haz-Map(R). All rights reserved. Unless otherwise indicated, all materials from Haz-Map are copyrighted by Haz-Map(R). No part of these materials, either text or image may be used for any purpose other than for personal use. Therefore, reproduction, modification, storage in a retrieval system or retransmission, in any form or by any means, electronic, mechanical or otherwise, for reasons other than personal use, is strictly prohibited without prior written permission. | |
CAS No. |
14630-40-1 | |
Record name | 1,1′-(1,2-Ethynediyl)bis[1,1,1-trimethylsilane] | |
Source | CAS Common Chemistry | |
URL | https://commonchemistry.cas.org/detail?cas_rn=14630-40-1 | |
Description | CAS Common Chemistry is an open community resource for accessing chemical information. Nearly 500,000 chemical substances from CAS REGISTRY cover areas of community interest, including common and frequently regulated chemicals, and those relevant to high school and undergraduate chemistry classes. This chemical information, curated by our expert scientists, is provided in alignment with our mission as a division of the American Chemical Society. | |
Explanation | The data from CAS Common Chemistry is provided under a CC-BY-NC 4.0 license, unless otherwise stated. | |
Record name | Silane, 1,1'-(1,2-ethynediyl)bis(1,1,1-trimethyl- | |
Source | ChemIDplus | |
URL | https://pubchem.ncbi.nlm.nih.gov/substance/?source=chemidplus&sourceid=0014630401 | |
Description | ChemIDplus is a free, web search system that provides access to the structure and nomenclature authority files used for the identification of chemical substances cited in National Library of Medicine (NLM) databases, including the TOXNET system. | |
Record name | Silane, 1,1'-(1,2-ethynediyl)bis[1,1,1-trimethyl- | |
Source | EPA Chemicals under the TSCA | |
URL | https://www.epa.gov/chemicals-under-tsca | |
Description | EPA Chemicals under the Toxic Substances Control Act (TSCA) collection contains information on chemicals and their regulations under TSCA, including non-confidential content from the TSCA Chemical Substance Inventory and Chemical Data Reporting. | |
Record name | Bis(trimethylsilyl)acetylene | |
Source | EPA DSSTox | |
URL | https://comptox.epa.gov/dashboard/DTXSID3065798 | |
Description | DSSTox provides a high quality public chemistry resource for supporting improved predictive toxicology. | |
Record name | Bis(trimethylsilyl)acetylene | |
Source | European Chemicals Agency (ECHA) | |
URL | https://echa.europa.eu/substance-information/-/substanceinfo/100.035.141 | |
Description | The European Chemicals Agency (ECHA) is an agency of the European Union which is the driving force among regulatory authorities in implementing the EU's groundbreaking chemicals legislation for the benefit of human health and the environment as well as for innovation and competitiveness. | |
Explanation | Use of the information, documents and data from the ECHA website is subject to the terms and conditions of this Legal Notice, and subject to other binding limitations provided for under applicable law, the information, documents and data made available on the ECHA website may be reproduced, distributed and/or used, totally or in part, for non-commercial purposes provided that ECHA is acknowledged as the source: "Source: European Chemicals Agency, http://echa.europa.eu/". Such acknowledgement must be included in each copy of the material. ECHA permits and encourages organisations and individuals to create links to the ECHA website under the following cumulative conditions: Links can only be made to webpages that provide a link to the Legal Notice page. | |
Retrosynthesis Analysis
AI-Powered Synthesis Planning: Our tool employs the Template_relevance Pistachio, Template_relevance Bkms_metabolic, Template_relevance Pistachio_ringbreaker, Template_relevance Reaxys, Template_relevance Reaxys_biocatalysis model, leveraging a vast database of chemical reactions to predict feasible synthetic routes.
One-Step Synthesis Focus: Specifically designed for one-step synthesis, it provides concise and direct routes for your target compounds, streamlining the synthesis process.
Accurate Predictions: Utilizing the extensive PISTACHIO, BKMS_METABOLIC, PISTACHIO_RINGBREAKER, REAXYS, REAXYS_BIOCATALYSIS database, our tool offers high-accuracy predictions, reflecting the latest in chemical research and data.
Strategy Settings
Precursor scoring | Relevance Heuristic |
---|---|
Min. plausibility | 0.01 |
Model | Template_relevance |
Template Set | Pistachio/Bkms_metabolic/Pistachio_ringbreaker/Reaxys/Reaxys_biocatalysis |
Top-N result to add to graph | 6 |
Feasible Synthetic Routes
Disclaimer and Information on In-Vitro Research Products
Please be aware that all articles and product information presented on BenchChem are intended solely for informational purposes. The products available for purchase on BenchChem are specifically designed for in-vitro studies, which are conducted outside of living organisms. In-vitro studies, derived from the Latin term "in glass," involve experiments performed in controlled laboratory settings using cells or tissues. It is important to note that these products are not categorized as medicines or drugs, and they have not received approval from the FDA for the prevention, treatment, or cure of any medical condition, ailment, or disease. We must emphasize that any form of bodily introduction of these products into humans or animals is strictly prohibited by law. It is essential to adhere to these guidelines to ensure compliance with legal and ethical standards in research and experimentation.