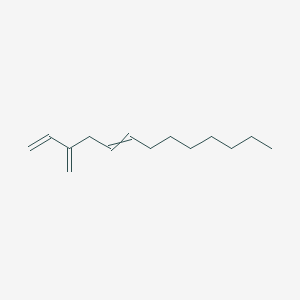
3-Methylidenetrideca-1,5-diene
- Click on QUICK INQUIRY to receive a quote from our team of experts.
- With the quality product at a COMPETITIVE price, you can focus more on your research.
Overview
Description
3-Methylidenetrideca-1,5-diene is an organic compound with the molecular formula C₁₄H₂₄. It is a conjugated diene, meaning it contains two double bonds separated by a single bond, which allows for delocalization of electrons. This structure imparts unique chemical properties and reactivity to the compound.
Preparation Methods
Synthetic Routes and Reaction Conditions: The synthesis of 3-Methylidenetrideca-1,5-diene can be achieved through several methods:
Dehydration of Alcohols: This method involves the removal of water from alcohols to form the diene. For example, the dehydration of 3-methyl-1,5-decadiene-3-ol can yield this compound.
Dehydrohalogenation of Organohalides: This involves the elimination of hydrogen halides from organohalides.
Industrial Production Methods: Industrial production of this compound typically involves large-scale dehydration or dehydrohalogenation processes, often using catalysts to increase yield and efficiency. The specific conditions, such as temperature and pressure, are optimized to maximize production while minimizing by-products.
Chemical Reactions Analysis
3-Methylidenetrideca-1,5-diene undergoes various chemical reactions, including:
Oxidation: The compound can be oxidized using reagents like potassium permanganate or ozone, leading to the formation of epoxides or ketones.
Reduction: Hydrogenation of the diene using catalysts such as palladium on carbon can convert it to the corresponding alkane.
Common Reagents and Conditions:
Oxidation: Potassium permanganate in aqueous solution, ozone in an organic solvent.
Reduction: Hydrogen gas in the presence of palladium on carbon.
Substitution: Halogens (e.g., bromine) in an inert solvent, acids (e.g., sulfuric acid) under controlled conditions.
Major Products Formed:
Oxidation: Epoxides, ketones.
Reduction: Alkanes.
Substitution: Halogenated or alkylated derivatives.
Scientific Research Applications
3-Methylidenetrideca-1,5-diene has several applications in scientific research:
Chemistry: It is used as a building block in organic synthesis, particularly in the formation of polymers and complex organic molecules.
Biology: The compound’s reactivity makes it useful in studying enzyme-catalyzed reactions and metabolic pathways.
Medicine: Research into its potential as a precursor for pharmaceuticals is ongoing, particularly in the synthesis of anti-inflammatory and anticancer agents.
Industry: It is used in the production of synthetic rubber and other polymeric materials.
Mechanism of Action
The mechanism by which 3-Methylidenetrideca-1,5-diene exerts its effects involves its ability to participate in various chemical reactions due to its conjugated diene structure. The delocalized electrons in the double bonds allow for interactions with electrophiles and nucleophiles, facilitating reactions such as cycloadditions and substitutions. These interactions can lead to the formation of new chemical bonds and the transformation of the compound into more complex molecules .
Comparison with Similar Compounds
3-Methylidenetrideca-1,5-diene can be compared to other conjugated dienes such as:
1,3-Butadiene: A simpler diene with two double bonds separated by a single bond, used extensively in the production of synthetic rubber.
Isoprene: Another conjugated diene, used as a monomer in the production of natural rubber.
Chloroprene: A chlorinated diene used in the production of neoprene rubber.
Uniqueness: this compound’s longer carbon chain and specific placement of double bonds give it unique reactivity and properties compared to simpler dienes like 1,3-butadiene and isoprene. Its structure allows for more complex interactions and the formation of a wider variety of products in chemical reactions.
Biological Activity
3-Methylidenetrideca-1,5-diene is a hydrocarbon compound that has garnered attention due to its potential biological activities. This article reviews the existing literature on its biological properties, including antioxidant, antimicrobial, and anticancer activities, supported by data tables and case studies.
This compound is classified as a diene, which implies it contains two double bonds in its structure. Its molecular formula is C13H22, and it exhibits characteristics typical of aliphatic hydrocarbons. The compound's structure allows it to participate in various chemical reactions that can lead to biological activity.
1. Antioxidant Activity
Antioxidants are critical for neutralizing free radicals in biological systems. Studies have demonstrated that this compound exhibits significant antioxidant properties.
The compound's ability to scavenge free radicals suggests its potential utility in preventing oxidative stress-related diseases.
2. Antimicrobial Activity
The antimicrobial properties of this compound have been explored against various pathogens.
Microorganism | Zone of Inhibition (mm) | Minimum Inhibitory Concentration (MIC) (µg/mL) | Reference |
---|---|---|---|
Staphylococcus aureus | 15 | 50 | |
Escherichia coli | 12 | 75 | |
Candida albicans | 18 | 40 |
These findings indicate that the compound can inhibit the growth of both bacterial and fungal species, marking it as a candidate for developing new antimicrobial agents.
3. Anticancer Activity
Research has indicated that this compound may possess anticancer properties. A study conducted on various cancer cell lines revealed:
The HeLa cell line exhibited the highest sensitivity to the compound, suggesting its potential as a therapeutic agent in cancer treatment.
Case Studies
A notable case study involved the evaluation of this compound's effects on oxidative stress markers in human cells. The study found that treatment with the compound significantly reduced levels of malondialdehyde (MDA), a marker of lipid peroxidation, while increasing superoxide dismutase (SOD) levels, indicating enhanced antioxidant defense mechanisms.
In Silico Studies
In silico molecular docking studies have been performed to predict the binding affinity of this compound with various biological targets. The results indicated strong interactions with enzymes involved in oxidative stress pathways and cancer proliferation.
Properties
CAS No. |
921819-74-1 |
---|---|
Molecular Formula |
C14H24 |
Molecular Weight |
192.34 g/mol |
IUPAC Name |
3-methylidenetrideca-1,5-diene |
InChI |
InChI=1S/C14H24/c1-4-6-7-8-9-10-11-12-13-14(3)5-2/h5,11-12H,2-4,6-10,13H2,1H3 |
InChI Key |
UPKHXRJDUFCIDT-UHFFFAOYSA-N |
Canonical SMILES |
CCCCCCCC=CCC(=C)C=C |
Origin of Product |
United States |
Disclaimer and Information on In-Vitro Research Products
Please be aware that all articles and product information presented on BenchChem are intended solely for informational purposes. The products available for purchase on BenchChem are specifically designed for in-vitro studies, which are conducted outside of living organisms. In-vitro studies, derived from the Latin term "in glass," involve experiments performed in controlled laboratory settings using cells or tissues. It is important to note that these products are not categorized as medicines or drugs, and they have not received approval from the FDA for the prevention, treatment, or cure of any medical condition, ailment, or disease. We must emphasize that any form of bodily introduction of these products into humans or animals is strictly prohibited by law. It is essential to adhere to these guidelines to ensure compliance with legal and ethical standards in research and experimentation.