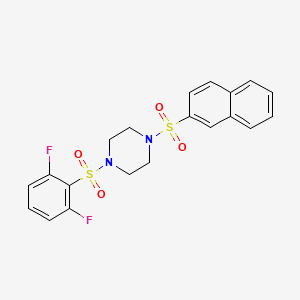
PKM2 activator 2
Overview
Description
PKM2 activator 2 is a chemical compound known for its ability to activate pyruvate kinase M2 (PKM2), an enzyme that plays a crucial role in glycolysis. This compound has shown potential in restoring normal glycolytic metabolism in cells, making it a significant compound in cancer research and metabolic studies .
Preparation Methods
Synthetic Routes and Reaction Conditions
The synthesis of PKM2 activator 2 involves multiple steps, typically starting with the preparation of key intermediates. The process often includes reactions such as nucleophilic substitution, condensation, and cyclization. Specific reaction conditions, such as temperature, solvent, and catalysts, are optimized to achieve high yield and purity. For instance, the use of dimethyl sulfoxide (DMSO) as a solvent and specific catalysts can enhance the reaction efficiency .
Industrial Production Methods
Industrial production of this compound requires scaling up the laboratory synthesis methods while ensuring consistency and quality. This involves using large-scale reactors, precise control of reaction parameters, and rigorous purification processes. Techniques such as crystallization, chromatography, and recrystallization are employed to obtain the final product with high purity .
Chemical Reactions Analysis
Types of Reactions
PKM2 activator 2 undergoes various chemical reactions, including:
Oxidation: This reaction involves the addition of oxygen or the removal of hydrogen, often using oxidizing agents like hydrogen peroxide or potassium permanganate.
Reduction: This involves the gain of electrons or hydrogen, typically using reducing agents such as sodium borohydride or lithium aluminum hydride.
Substitution: This reaction involves the replacement of one functional group with another, often using reagents like halogens or nucleophiles.
Common Reagents and Conditions
Common reagents used in the reactions of this compound include:
Oxidizing agents: Hydrogen peroxide, potassium permanganate.
Reducing agents: Sodium borohydride, lithium aluminum hydride.
Nucleophiles: Halogens, amines, and other nucleophilic species.
Major Products
The major products formed from these reactions depend on the specific conditions and reagents used. For example, oxidation reactions may yield oxidized derivatives, while reduction reactions produce reduced forms of the compound .
Scientific Research Applications
Applications in Cancer Research
-
Tumor Suppression
- Study Findings : Research has shown that PKM2 activators can suppress tumor formation by enhancing PKM2 activity. For instance, the compound DASA-58 was found to increase glucose consumption and lactate secretion in lung cancer cells, indicating a shift towards aerobic glycolysis, which is often utilized by cancer cells for rapid growth .
- Case Study : In experiments with human cancer cell xenografts, continuous dosing with PKM2 activators significantly decreased tumor development, suggesting that these compounds can impair tumorigenesis through metabolic reprogramming .
-
Sensitization to Chemotherapy
- Mechanism : Activation of PKM2 has been linked to increased sensitivity of cancer cells to various chemotherapeutic agents. For example, silencing PKM2 expression enhances the efficacy of cisplatin in non-small cell lung cancer models .
- Research Insight : A study demonstrated that PKM2 activation could sensitize tumor-initiating cells (TICs) to metabolic perturbations, leading to inhibited growth under conventional culture conditions .
-
Metabolic Regulation
- Aerobic Glycolysis : Activation of PKM2 alters cellular metabolism by promoting aerobic glycolysis, which is essential for cancer cell proliferation. This was evidenced by increased glucose uptake and lactate production in treated cells compared to controls .
- Impact on Cell Viability : Interestingly, while PKM2 activation enhances metabolic activity, it does not necessarily correlate with increased cell viability under all conditions, highlighting the complex role of PKM2 in cancer biology .
Applications in Metabolic Diseases
- Kidney Disease
- Emerging Research : Recent studies have indicated that PKM2 plays a role in kidney disease progression, particularly in diabetic conditions. Activators like PKM2 activator 2 may offer therapeutic potential by restoring normal metabolic functions within renal cells .
- Clinical Implications : The modulation of PKM2 activity could lead to novel treatment strategies for managing kidney diseases, with ongoing research focused on understanding its mechanisms and effects on renal metabolism .
Summary Table of Key Findings
Mechanism of Action
PKM2 activator 2 exerts its effects by activating pyruvate kinase M2, which is a key enzyme in the glycolytic pathway. By activating PKM2, the compound enhances the conversion of phosphoenolpyruvate to pyruvate, leading to increased glycolytic flux. This activation helps restore normal glycolytic metabolism, which is often disrupted in cancer cells. The molecular targets and pathways involved include the regulation of gene expression related to apoptosis, mitosis, hypoxia, inflammation, and metabolic reprogramming .
Comparison with Similar Compounds
Similar Compounds
PKM2 activator 1: Another activator of pyruvate kinase M2, but with different potency and specificity.
PKM2 activator 3: A compound with similar activating properties but distinct chemical structure and pharmacokinetics.
Uniqueness
PKM2 activator 2 is unique due to its high potency and specificity for PKM2, with an AC50 value of 66 nM. This makes it a highly effective tool for studying and modulating glycolytic metabolism in various research contexts .
Biological Activity
Pyruvate kinase M2 (PKM2) is a critical enzyme in the glycolytic pathway, playing a significant role in cellular metabolism, particularly in cancer cells. The activation of PKM2 is associated with various biological processes, including tumorigenesis, immune response modulation, and metabolic reprogramming. PKM2 activator 2 (often referred to as DASA-58 or TEPP-46) has emerged as a promising small-molecule compound that enhances the enzymatic activity of PKM2. This article delves into the biological activity of this compound, supported by research findings, data tables, and relevant case studies.
PKM2 exists in two main forms: a high-activity tetramer and a low-activity dimer/monomer. Activators like DASA-58 stabilize the tetrameric form, enhancing its catalytic efficiency. The binding of these activators occurs at the interface of the PKM2 subunits, promoting their association into stable tetramers and rendering the enzyme resistant to inhibitory modifications induced by tyrosine phosphorylation .
Key Findings:
- Tetramer Stabilization : DASA-58 increases PKM2 activity in a dose-dependent manner, achieving an effective half-activation concentration (EC50) of approximately 19.6 µM .
- Resistance to Inhibition : Pre-treatment with DASA-58 prevents the inhibition of PKM2 activity caused by pervanadate treatment, which induces tyrosine phosphorylation .
Biological Implications
The activation of PKM2 has significant implications for various biological processes:
1. Cancer Metabolism
PKM2 activators have shown potential in suppressing tumor growth. For instance, in preclinical studies involving human cancer cell xenografts, continuous dosing with TEPP-46 resulted in reduced tumor development . The activation of PKM2 leads to increased aerobic glycolysis and lactate secretion, which are metabolic characteristics commonly associated with cancer cells .
2. Immune Response Modulation
Recent studies indicate that PKM2 activators can enhance macrophage function by promoting mitochondrial biogenesis and endotoxin tolerance. TEPP-46 treatment increased the expression of pro-inflammatory cytokines and contributed to metabolic reprogramming within macrophages . This suggests that targeting PKM2 could be a novel therapeutic strategy for treating inflammatory diseases.
Case Studies
Several case studies highlight the efficacy and mechanisms of action of PKM2 activators:
Case Study 1: TEPP-46 and Macrophage Function
A study demonstrated that TEPP-46 treatment enhanced mitochondrial biogenesis in macrophages, leading to improved endotoxin tolerance. Mice treated with TEPP-46 exhibited higher levels of the PKM2 tetramer and reduced inflammatory responses after lipopolysaccharide (LPS) exposure .
Case Study 2: DASA-58 in Cancer Therapy
In vitro experiments showed that DASA-58 significantly increased glucose consumption and lactate production in H1299 lung cancer cells compared to control treatments. This metabolic shift was linked to enhanced cell viability under nutrient-deprived conditions, suggesting a potential therapeutic role for DASA-58 in cancer metabolism .
Data Tables
Compound | EC50 (µM) | Effect on Tumor Growth | Mechanism |
---|---|---|---|
DASA-58 | 19.6 | Reduced tumor development | Tetramer stabilization |
TEPP-46 | N/A | Enhanced macrophage function | Mitochondrial biogenesis |
Q & A
Basic Research Questions
Q. What is the molecular mechanism by which PKM2 activator 2 modulates enzyme activity, and how is this validated experimentally?
this compound binds to an allosteric site on the PKM2 enzyme, promoting its transition from an inactive dimeric state to an active tetrameric conformation. Structural studies using X-ray crystallography reveal critical interactions with residues such as L394 and Q393 via hydrogen bonding and hydrophobic contacts . Experimental validation includes immunoprecipitation (IP) and Western blotting to confirm tetramer formation, as well as luciferase-coupled assays to quantify enzymatic activation (AC50 = 66 nM) .
Q. How does this compound address the Warburg effect in cancer metabolism?
The Warburg effect describes the preference of cancer cells for aerobic glycolysis over oxidative phosphorylation. This compound normalizes glycolytic flux by restoring PKM2 tetramer activity, which reduces lactate production and redirects metabolic intermediates toward oxidative phosphorylation. This shift is validated via extracellular acidification rate (ECAR) and oxygen consumption rate (OCR) assays in cancer cell lines .
Q. What in vitro assays are most appropriate for assessing PKM2 activation efficacy?
Key assays include:
- Luciferase-coupled enzymatic assays to measure PKM2 activity (AC50 determination) .
- Immunoblotting to detect tetramer:monomer ratios using anti-PKM2 antibodies .
- Metabolomic profiling (e.g., LC-MS) to quantify glycolytic intermediates (e.g., phosphoenolpyruvate, lactate) . Controls should include wild-type vs. PKM2-knockout cells and structurally unrelated activators (e.g., TEPP-46) to confirm specificity .
Advanced Research Questions
Q. How can researchers resolve contradictory findings on this compound’s effects across cancer models?
Discrepancies may arise from cell-type-specific metabolic dependencies or genetic backgrounds. For example, this compound induces apoptosis in colorectal cancer cells (e.g., DLD-1, IC50 = 10.04 µM) but primarily inhibits proliferation in lung cancer models (e.g., A549) . To address this, researchers should:
- Perform transcriptomic profiling to identify baseline metabolic pathway activation.
- Use isogenic cell lines (e.g., PKM2 wild-type vs. knockdown) to isolate compound-specific effects.
- Validate findings in 3D spheroid or patient-derived xenograft (PDX) models to better mimic tumor heterogeneity .
Q. What experimental strategies mitigate off-target effects of this compound in metabolic studies?
Off-target effects can be minimized by:
- Dose-response titrations to establish the therapeutic window (e.g., comparing AC50 values with cytotoxicity thresholds) .
- Chemical proteomics (e.g., affinity chromatography coupled with mass spectrometry) to identify unintended binding partners.
- Genetic validation using PKM2-specific siRNA or CRISPR-Cas9 to confirm phenotype reversibility .
Q. How should researchers design experiments to evaluate this compound in combination therapies?
Rational combinations might target complementary pathways, such as:
- AMPK activators (e.g., AMPK activator 2) to enhance energy stress .
- PDK1 inhibitors to further suppress glycolytic flux . Experimental design should include:
- Synergy analysis via Chou-Talalay isobolograms.
- Longitudinal metabolomics to track dynamic metabolic adaptations.
- In vivo efficacy studies using orthotopic tumor models to assess pharmacokinetic compatibility .
Q. What are the critical controls for validating this compound’s role in proteasome-mediated pathways?
Recent studies suggest PKM2 overexpression attenuates proteasome-mediated degradation of proteins like PHB2 in cardiomyocytes . Key controls include:
- Proteasome inhibitors (e.g., MG132) to confirm degradation pathway involvement.
- Pulse-chase assays to measure protein half-life changes.
- Mutant PKM2 constructs (e.g., K305Q) to dissect enzymatic vs. non-catalytic roles .
Q. Methodological Notes
- Structural Validation : Always cross-reference biochemical data with structural insights (e.g., co-crystallization studies) to confirm binding modes .
- Data Reproducibility : Adhere to guidelines for experimental reporting (e.g., Beilstein Journal of Organic Chemistry’s protocols for compound characterization) .
- Ethical Compliance : Ensure animal or human-derived models follow institutional review board (IRB) protocols, particularly for PDX studies .
Properties
Molecular Formula |
C20H18F2N2O4S2 |
---|---|
Molecular Weight |
452.5 g/mol |
IUPAC Name |
1-(2,6-difluorophenyl)sulfonyl-4-naphthalen-2-ylsulfonylpiperazine |
InChI |
InChI=1S/C20H18F2N2O4S2/c21-18-6-3-7-19(22)20(18)30(27,28)24-12-10-23(11-13-24)29(25,26)17-9-8-15-4-1-2-5-16(15)14-17/h1-9,14H,10-13H2 |
InChI Key |
LLOHMBFPOYWAIL-UHFFFAOYSA-N |
SMILES |
C1CN(CCN1S(=O)(=O)C2=CC3=CC=CC=C3C=C2)S(=O)(=O)C4=C(C=CC=C4F)F |
Canonical SMILES |
C1CN(CCN1S(=O)(=O)C2=CC3=CC=CC=C3C=C2)S(=O)(=O)C4=C(C=CC=C4F)F |
Origin of Product |
United States |
Retrosynthesis Analysis
AI-Powered Synthesis Planning: Our tool employs the Template_relevance Pistachio, Template_relevance Bkms_metabolic, Template_relevance Pistachio_ringbreaker, Template_relevance Reaxys, Template_relevance Reaxys_biocatalysis model, leveraging a vast database of chemical reactions to predict feasible synthetic routes.
One-Step Synthesis Focus: Specifically designed for one-step synthesis, it provides concise and direct routes for your target compounds, streamlining the synthesis process.
Accurate Predictions: Utilizing the extensive PISTACHIO, BKMS_METABOLIC, PISTACHIO_RINGBREAKER, REAXYS, REAXYS_BIOCATALYSIS database, our tool offers high-accuracy predictions, reflecting the latest in chemical research and data.
Strategy Settings
Precursor scoring | Relevance Heuristic |
---|---|
Min. plausibility | 0.01 |
Model | Template_relevance |
Template Set | Pistachio/Bkms_metabolic/Pistachio_ringbreaker/Reaxys/Reaxys_biocatalysis |
Top-N result to add to graph | 6 |
Feasible Synthetic Routes
Disclaimer and Information on In-Vitro Research Products
Please be aware that all articles and product information presented on BenchChem are intended solely for informational purposes. The products available for purchase on BenchChem are specifically designed for in-vitro studies, which are conducted outside of living organisms. In-vitro studies, derived from the Latin term "in glass," involve experiments performed in controlled laboratory settings using cells or tissues. It is important to note that these products are not categorized as medicines or drugs, and they have not received approval from the FDA for the prevention, treatment, or cure of any medical condition, ailment, or disease. We must emphasize that any form of bodily introduction of these products into humans or animals is strictly prohibited by law. It is essential to adhere to these guidelines to ensure compliance with legal and ethical standards in research and experimentation.