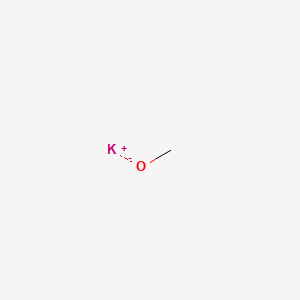
Potassium methoxide
- Click on QUICK INQUIRY to receive a quote from our team of experts.
- With the quality product at a COMPETITIVE price, you can focus more on your research.
Overview
Description
Potassium methoxide, also known as this compound, is a useful research compound. Its molecular formula is CH4KO and its molecular weight is 71.140 g/mol. The purity is usually 95%.
The exact mass of the compound Potassium methanolate is unknown and the complexity rating of the compound is unknown. The solubility of this chemical has been described as solubility in water: reaction. The United Nations designated GHS hazard class pictogram is Flammable;Corrosive;Irritant, and the GHS signal word is DangerThe storage condition is unknown. Please store according to label instructions upon receipt of goods.
BenchChem offers high-quality this compound suitable for many research applications. Different packaging options are available to accommodate customers' requirements. Please inquire for more information about this compound including the price, delivery time, and more detailed information at info@benchchem.com.
Scientific Research Applications
Biodiesel Production
Potassium methanolate plays a significant role in the production of biodiesel. Research has focused on optimizing the biodiesel production process, particularly from waste cooking oil. It is used as a catalyst in transesterification, a chemical process converting triglycerides into biodiesel and glycerol. Studies demonstrate that potassium methanolate aids in achieving high yields of biodiesel with optimal energy efficiency and purity (Mostafaei et al., 2015), (Vicente et al., 2007), (Tomašević & Šiler-Marinković, 2003).
Methanol Synthesis
Potassium methanolate is also involved in methanol synthesis. It has been found to be a promoter for methanol synthesis from CO + H2, indicating its potential in industrial applications for synthesizing methanol (Maack et al., 2003).
Hydrogen-Rich Gas Generation
In the context of renewable energy, potassium methanolate is used in the steam reforming of ethanol and methanol. This process generates hydrogen-rich gas, which can be used in fuel cells. The research has examined the effects of potassium on catalyst activity and selectivity, emphasizing its efficiency in hydrogen generation (Machocki et al., 2016).
Chemical Interesterification
Potassium methanolate is used in the chemical interesterification of oils, such as sunflower oil, with methyl acetate. This process modifies the oil's physical properties, making it suitable for various industrial applications, including biodiesel production (Casas et al., 2011).
Electrochemical Reactions
Potassium methanolate contributes to electrochemical reactions, such as the C-H methylthiolation of electron-rich aromatics. This process has applications in synthetic chemistry, offering a metal-free and environmentally friendly method for functionalizing aromatic compounds (Wu et al., 2020).
Mechanism of Action
Target of Action
Potassium methoxide, also known as potassium methanolate, is primarily used as a strong base and a catalyst for transesterification . Its primary targets are the molecules involved in the transesterification process, such as triglycerides in the production of biodiesel .
Mode of Action
This compound acts as a basic nucleophile, facilitating the dehalogenation of arylhalides . It also serves as a methoxylating agent for the methoxylation of bromo- and mesyloxyalkanes . As a catalyst, it accelerates the transesterification process, particularly in the production of biodiesel .
Biochemical Pathways
The compound plays a crucial role in the transesterification process, a key biochemical pathway in the production of biodiesel . This process involves the reaction of triglycerides with methanol (or another short-chained alcohol) to produce esters and glycerol . This compound facilitates this reaction, leading to the efficient production of biodiesel .
Pharmacokinetics
It’s important to note that this compound is a highly reactive compound that reacts violently with water, forming potassium hydroxide and methanol . This reactivity needs to be carefully managed in industrial settings.
Result of Action
The primary result of this compound’s action is the efficient production of biodiesel through the transesterification process . It also facilitates the formation of fatty soaps, with higher yields obtained compared to using sodium methoxide .
Action Environment
The action of this compound is influenced by environmental factors. For instance, it is hygroscopic and reacts violently with water . Therefore, it must be handled in an environment with controlled moisture levels. Additionally, it is a white to yellowish, odorless crystalline powder, and its solutions are highly basic and have a corrosive effect . These properties necessitate careful handling and storage procedures to ensure its stability and efficacy.
Safety and Hazards
Potassium methanolate is highly flammable and toxic if swallowed or in contact with skin . It causes severe skin burns and eye damage, and may cause respiratory irritation . It is toxic if inhaled and causes damage to organs . The solid is very hygroscopic and decomposes quickly. It is only stable under exclusion of air and moisture .
Future Directions
The future demand for potassium methanolate is expected to be driven by specific factors, such as technological advancements, changing consumer behaviors, regulatory shifts, or global trends . As the market evolves, several key players will likely emerge as influential forces . Among the top contenders are leading companies or organizations, known for their innovation, market presence, and strategic initiatives .
Biochemical Analysis
Biochemical Properties
Potassium methanolate plays a significant role in biochemical reactions due to its strong basic nature. It interacts with various enzymes, proteins, and other biomolecules, primarily through deprotonation reactions. For instance, potassium methanolate can deprotonate alcohols, phenols, and carboxylic acids, making it a valuable reagent in organic synthesis. The compound’s ability to act as a strong base allows it to facilitate the formation of enolates from carbonyl compounds, which are crucial intermediates in many biochemical pathways .
Cellular Effects
Potassium methanolate influences various cellular processes by altering the pH and ionic balance within cells. It can affect cell signaling pathways, gene expression, and cellular metabolism. For example, the compound’s strong basic nature can lead to the deprotonation of cellular components, disrupting normal cellular functions. Additionally, potassium methanolate can interfere with the activity of enzymes that are pH-sensitive, leading to changes in metabolic flux and gene expression .
Molecular Mechanism
At the molecular level, potassium methanolate exerts its effects through deprotonation and nucleophilic substitution reactions. The compound can bind to and deprotonate acidic protons in biomolecules, leading to the formation of reactive intermediates. These intermediates can then participate in various biochemical reactions, such as the formation of carbon-carbon bonds or the cleavage of ester bonds. Potassium methanolate can also inhibit or activate enzymes by altering their active sites through deprotonation .
Temporal Effects in Laboratory Settings
In laboratory settings, the effects of potassium methanolate can change over time due to its stability and degradation. The compound is hygroscopic and can absorb moisture from the air, leading to the formation of potassium hydroxide and methanol. This degradation can affect the compound’s reactivity and long-term effects on cellular function. In in vitro and in vivo studies, the stability of potassium methanolate is crucial for maintaining consistent experimental conditions .
Dosage Effects in Animal Models
The effects of potassium methanolate vary with different dosages in animal models. At low doses, the compound can act as a mild base, facilitating biochemical reactions without causing significant toxicity. At high doses, potassium methanolate can lead to adverse effects, such as tissue damage and disruption of normal cellular functions. Threshold effects are observed, where the compound’s impact on cellular processes becomes more pronounced at higher concentrations .
Metabolic Pathways
Potassium methanolate is involved in various metabolic pathways, primarily through its role as a strong base. It interacts with enzymes and cofactors that participate in deprotonation and nucleophilic substitution reactions. The compound can affect metabolic flux by altering the availability of reactive intermediates and influencing the activity of key enzymes. For example, potassium methanolate can facilitate the formation of enolates, which are important intermediates in gluconeogenesis and other metabolic pathways .
Transport and Distribution
Within cells and tissues, potassium methanolate is transported and distributed based on its ionic nature. The compound can interact with transporters and binding proteins that facilitate its movement across cellular membranes. Potassium methanolate’s localization and accumulation can affect its reactivity and impact on cellular processes. For instance, the compound’s distribution within specific cellular compartments can influence its ability to deprotonate biomolecules and participate in biochemical reactions .
Subcellular Localization
Potassium methanolate’s subcellular localization is influenced by its chemical properties and interactions with cellular components. The compound can be directed to specific compartments or organelles through targeting signals or post-translational modifications. Its activity and function can be affected by its localization, as the local pH and ionic environment can influence its reactivity. For example, potassium methanolate’s presence in the mitochondria can impact mitochondrial metabolism and energy production .
Properties
{ "Design of the Synthesis Pathway": "The synthesis of Potassium methanolate can be achieved by reacting potassium hydroxide with methanol.", "Starting Materials": ["Potassium hydroxide", "Methanol"], "Reaction": [ "Add 20g of potassium hydroxide to a 250 mL round bottom flask.", "Add 100 mL of methanol to the flask and stir until the potassium hydroxide dissolves completely.", "Heat the mixture to reflux for 4 hours, stirring occasionally.", "Allow the mixture to cool to room temperature.", "Filter the mixture through a filter paper to remove any solid impurities.", "Collect the filtrate in a clean, dry flask.", "Evaporate the solvent under reduced pressure using a rotary evaporator.", "Dry the resulting solid in a vacuum desiccator over anhydrous calcium chloride.", "The resulting product is Potassium methanolate." ] } | |
CAS No. |
865-33-8 |
Molecular Formula |
CH4KO |
Molecular Weight |
71.140 g/mol |
IUPAC Name |
potassium;methanolate |
InChI |
InChI=1S/CH4O.K/c1-2;/h2H,1H3; |
InChI Key |
VXJPCEOTZNHHOA-UHFFFAOYSA-N |
SMILES |
C[O-].[K+] |
Canonical SMILES |
CO.[K] |
density |
1.7 g/cm³ |
flash_point |
11 °C c.c. |
melting_point |
No melting point; decomposes at >50 °C |
865-33-8 | |
physical_description |
Liquid WHITE-TO-YELLOW HYGROSCOPIC POWDER. |
Pictograms |
Flammable; Corrosive; Irritant |
solubility |
Solubility in water: reaction |
Synonyms |
potassium methylate |
vapor_pressure |
Vapor pressure, Pa at 25 °C: (negligible) |
Origin of Product |
United States |
Retrosynthesis Analysis
AI-Powered Synthesis Planning: Our tool employs the Template_relevance Pistachio, Template_relevance Bkms_metabolic, Template_relevance Pistachio_ringbreaker, Template_relevance Reaxys, Template_relevance Reaxys_biocatalysis model, leveraging a vast database of chemical reactions to predict feasible synthetic routes.
One-Step Synthesis Focus: Specifically designed for one-step synthesis, it provides concise and direct routes for your target compounds, streamlining the synthesis process.
Accurate Predictions: Utilizing the extensive PISTACHIO, BKMS_METABOLIC, PISTACHIO_RINGBREAKER, REAXYS, REAXYS_BIOCATALYSIS database, our tool offers high-accuracy predictions, reflecting the latest in chemical research and data.
Strategy Settings
Precursor scoring | Relevance Heuristic |
---|---|
Min. plausibility | 0.01 |
Model | Template_relevance |
Template Set | Pistachio/Bkms_metabolic/Pistachio_ringbreaker/Reaxys/Reaxys_biocatalysis |
Top-N result to add to graph | 6 |
Feasible Synthetic Routes
Q1: What is the primary application of potassium methoxide in organic synthesis?
A1: this compound is widely recognized as a catalyst in transesterification reactions [, , , ]. This process is crucial for biodiesel production, where it facilitates the conversion of triglycerides from vegetable oils or animal fats into fatty acid methyl esters (FAME) [, , , ].
Q2: How does the catalytic activity of this compound compare to other alkaline catalysts in biodiesel production?
A2: Studies show that this compound exhibits superior catalytic activity compared to potassium hydroxide and sodium hydroxide in biodiesel production [, ]. It accelerates the reaction and leads to a higher conversion equilibrium, resulting in better biodiesel yields [, ].
Q3: Are there any drawbacks to using this compound as a catalyst in biodiesel production?
A3: While highly effective, this compound can lead to the formation of undesirable soap as a byproduct, especially when the feedstock contains free fatty acids [, ]. This can impact the purity and yield of the biodiesel produced.
Q4: Besides biodiesel production, what other applications utilize this compound's catalytic properties?
A4: this compound is a versatile catalyst employed in various other reactions. For example, it facilitates the dehalogenative deuteration of aryl halides, enabling the incorporation of deuterium into complex molecules for pharmaceutical and medicinal chemistry research []. It also catalyzes the anionic polymerization of lactones, leading to the synthesis of biodegradable polyesters [, ].
Q5: How does this compound promote dehalogenative deuteration reactions?
A5: In the presence of a deuterium source like CD3CN, this compound mediates the removal of a halogen atom from an aryl halide and its replacement with deuterium []. This method offers high deuterium incorporation and is particularly useful for labeling heteroarenes found in pharmaceuticals [].
Q6: Does this compound react with cellulose?
A6: Yes, this compound reacts with cellulose to form potassium cellulosates [, ]. This reaction is influenced by the concentration of this compound and can be enhanced by using co-solvents like dimethyl sulfoxide and pyridine [, ].
Q7: What is the significance of potassium cellulosate formation?
A7: Potassium cellulosates serve as valuable intermediates in the preparation of cellulose derivatives and can act as initiators for anionic graft polymerization of vinyl monomers [, ].
Q8: What is the molecular formula and weight of this compound?
A8: this compound has the molecular formula CH3KO and a molecular weight of 70.13 g/mol.
Q9: Does the cation associated with methoxide influence its reactivity?
A9: Yes, the counterion significantly affects methoxide reactivity. For instance, sodium methoxide exhibits higher basicity in methanol compared to this compound, particularly in reactions involving multi-charged adducts where stronger association with sodium ions is observed [].
Disclaimer and Information on In-Vitro Research Products
Please be aware that all articles and product information presented on BenchChem are intended solely for informational purposes. The products available for purchase on BenchChem are specifically designed for in-vitro studies, which are conducted outside of living organisms. In-vitro studies, derived from the Latin term "in glass," involve experiments performed in controlled laboratory settings using cells or tissues. It is important to note that these products are not categorized as medicines or drugs, and they have not received approval from the FDA for the prevention, treatment, or cure of any medical condition, ailment, or disease. We must emphasize that any form of bodily introduction of these products into humans or animals is strictly prohibited by law. It is essential to adhere to these guidelines to ensure compliance with legal and ethical standards in research and experimentation.