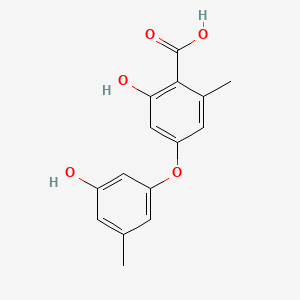
Deoxygerfelin
Overview
Description
Deoxygerfelin is a phenolic antioxidant that has been found in A. versicolor . It scavenges free radicals in a Trolox equivalent activity concentration (TEAC) assay .
Synthesis Analysis
The secondary metabolite 10-deoxygerfelin of A. sydowii acted as an inducer to induce B. subtilis to produce benzoic acid, which was further converted into 3-OH-benzoic acid by A. sydowii . Subsequently, A. sydowii used 3-OH-benzoic acid as the substrate to synthesize the new compound N2, and then N1, N3, N4, and N7 were biosynthesized upon the upregulation of hydrolase, hydroxylase, and acyltransferase during co-culture .Molecular Structure Analysis
The molecular formula of Deoxygerfelin is C15H14O5 . The exact mass is 274.08 and the molecular weight is 274.272 .Physical And Chemical Properties Analysis
Deoxygerfelin has a molecular formula of C15H14O5 and a formula weight of 274.3 .Scientific Research Applications
Antioxidant Research
Deoxygerfelin exhibits antioxidant properties by scavenging free radicals. This makes it valuable for research into oxidative stress and its effects on biological systems. Its efficacy can be measured using assays like the Trolox equivalent activity concentration (TEAC) assay .
Microbial Metabolite Studies
As a microbial metabolite, Deoxygerfelin’s role in the metabolism of microorganisms like A. versicolor can be studied. Researchers can explore how this compound is synthesized and regulated within fungal species .
Interspecific Interaction Studies
The compound plays a role in interspecific interactions, particularly in co-cultures. For example, Deoxygerfelin from A. sydowii can induce B. subtilis to produce novel benzoic acid derivatives, which are important for understanding microbial crosstalk .
Enzyme Regulation Research
Deoxygerfelin is involved in the regulation of enzymes during the co-culture of different microbial species. Studying its influence on enzyme activity can shed light on the biosynthesis of new compounds in microbial communities .
Secondary Metabolite Biosynthesis
Research into the biosynthesis pathways of secondary metabolites can be enhanced by studying Deoxygerfelin. It acts as a precursor or inducer in the synthesis of other compounds, providing insights into the complex biosynthetic processes in fungi .
Antimicrobial Activity Exploration
While not explicitly mentioned in the search results, phenolic antioxidants like Deoxygerfelin often exhibit antimicrobial properties. Investigating its potential antimicrobial activity could lead to the discovery of new antibiotics or preservatives .
Mechanism of Action
Target of Action
Deoxygerfelin is a phenolic antioxidant that has been found in A. versicolor . Its primary target is free radicals, which it scavenges in a Trolox equivalent activity concentration (TEAC) assay . Free radicals are unstable atoms that can cause damage to cells, contributing to aging and diseases. By scavenging these free radicals, Deoxygerfelin helps to prevent or slow down the damage to cells.
Safety and Hazards
properties
IUPAC Name |
2-hydroxy-4-(3-hydroxy-5-methylphenoxy)-6-methylbenzoic acid | |
---|---|---|
Source | PubChem | |
URL | https://pubchem.ncbi.nlm.nih.gov | |
Description | Data deposited in or computed by PubChem | |
InChI |
InChI=1S/C15H14O5/c1-8-3-10(16)6-11(4-8)20-12-5-9(2)14(15(18)19)13(17)7-12/h3-7,16-17H,1-2H3,(H,18,19) | |
Source | PubChem | |
URL | https://pubchem.ncbi.nlm.nih.gov | |
Description | Data deposited in or computed by PubChem | |
InChI Key |
ZJEIMBOWRAUNAN-UHFFFAOYSA-N | |
Source | PubChem | |
URL | https://pubchem.ncbi.nlm.nih.gov | |
Description | Data deposited in or computed by PubChem | |
Canonical SMILES |
CC1=CC(=CC(=C1)OC2=CC(=C(C(=C2)C)C(=O)O)O)O | |
Source | PubChem | |
URL | https://pubchem.ncbi.nlm.nih.gov | |
Description | Data deposited in or computed by PubChem | |
Molecular Formula |
C15H14O5 | |
Source | PubChem | |
URL | https://pubchem.ncbi.nlm.nih.gov | |
Description | Data deposited in or computed by PubChem | |
DSSTOX Substance ID |
DTXSID50635881 | |
Record name | 2-Hydroxy-4-(3-hydroxy-5-methylphenoxy)-6-methylbenzoic acid | |
Source | EPA DSSTox | |
URL | https://comptox.epa.gov/dashboard/DTXSID50635881 | |
Description | DSSTox provides a high quality public chemistry resource for supporting improved predictive toxicology. | |
Molecular Weight |
274.27 g/mol | |
Source | PubChem | |
URL | https://pubchem.ncbi.nlm.nih.gov | |
Description | Data deposited in or computed by PubChem | |
Product Name |
2-Hydroxy-4-(3-hydroxy-5-methylphenoxy)-6-methylbenzoic acid | |
CAS RN |
36149-01-6 | |
Record name | 4-Carboxydiorcinal | |
Source | CAS Common Chemistry | |
URL | https://commonchemistry.cas.org/detail?cas_rn=36149-01-6 | |
Description | CAS Common Chemistry is an open community resource for accessing chemical information. Nearly 500,000 chemical substances from CAS REGISTRY cover areas of community interest, including common and frequently regulated chemicals, and those relevant to high school and undergraduate chemistry classes. This chemical information, curated by our expert scientists, is provided in alignment with our mission as a division of the American Chemical Society. | |
Explanation | The data from CAS Common Chemistry is provided under a CC-BY-NC 4.0 license, unless otherwise stated. | |
Record name | 2-Hydroxy-4-(3-hydroxy-5-methylphenoxy)-6-methylbenzoic acid | |
Source | EPA DSSTox | |
URL | https://comptox.epa.gov/dashboard/DTXSID50635881 | |
Description | DSSTox provides a high quality public chemistry resource for supporting improved predictive toxicology. | |
Retrosynthesis Analysis
AI-Powered Synthesis Planning: Our tool employs the Template_relevance Pistachio, Template_relevance Bkms_metabolic, Template_relevance Pistachio_ringbreaker, Template_relevance Reaxys, Template_relevance Reaxys_biocatalysis model, leveraging a vast database of chemical reactions to predict feasible synthetic routes.
One-Step Synthesis Focus: Specifically designed for one-step synthesis, it provides concise and direct routes for your target compounds, streamlining the synthesis process.
Accurate Predictions: Utilizing the extensive PISTACHIO, BKMS_METABOLIC, PISTACHIO_RINGBREAKER, REAXYS, REAXYS_BIOCATALYSIS database, our tool offers high-accuracy predictions, reflecting the latest in chemical research and data.
Strategy Settings
Precursor scoring | Relevance Heuristic |
---|---|
Min. plausibility | 0.01 |
Model | Template_relevance |
Template Set | Pistachio/Bkms_metabolic/Pistachio_ringbreaker/Reaxys/Reaxys_biocatalysis |
Top-N result to add to graph | 6 |
Feasible Synthetic Routes
Q & A
Q1: How does deoxygerfelin influence the metabolic activity of Bacillus subtilis in co-culture?
A1: In co-culture, deoxygerfelin, a secondary metabolite produced by Aspergillus sydowii, acts as an inducer molecule for Bacillus subtilis. [] This induction stimulates B. subtilis to produce benzoic acid. This initial interaction triggers a cascade of metabolic events leading to the biosynthesis of novel benzoic acid derivatives. []
Q2: What is the significance of the interaction between Aspergillus sydowii and Bacillus subtilis mediated by deoxygerfelin?
A2: The deoxygerfelin-mediated interaction between A. sydowii and B. subtilis highlights the importance of interspecific crosstalk in microbial communities. [] This interaction leads to the production of novel benzoic acid derivatives, showcasing the potential of co-culture for discovering new bioactive compounds. [] This discovery has implications for understanding microbial ecology and exploring new avenues for drug discovery.
Disclaimer and Information on In-Vitro Research Products
Please be aware that all articles and product information presented on BenchChem are intended solely for informational purposes. The products available for purchase on BenchChem are specifically designed for in-vitro studies, which are conducted outside of living organisms. In-vitro studies, derived from the Latin term "in glass," involve experiments performed in controlled laboratory settings using cells or tissues. It is important to note that these products are not categorized as medicines or drugs, and they have not received approval from the FDA for the prevention, treatment, or cure of any medical condition, ailment, or disease. We must emphasize that any form of bodily introduction of these products into humans or animals is strictly prohibited by law. It is essential to adhere to these guidelines to ensure compliance with legal and ethical standards in research and experimentation.