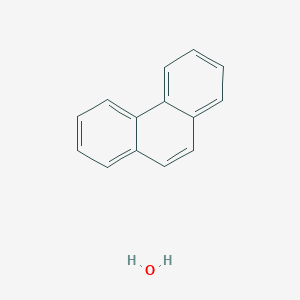
Phenanthrene water
- Click on QUICK INQUIRY to receive a quote from our team of experts.
- With the quality product at a COMPETITIVE price, you can focus more on your research.
Overview
Description
Phenanthrene (C₁₄H₁₀), a tricyclic polycyclic aromatic hydrocarbon (PAH), is a persistent environmental pollutant derived primarily from crude oil and incomplete combustion of organic matter . Its hydrophobic nature (log Kow ~4.5) and low water solubility (~1.1 mg/L at 25°C) contribute to its accumulation in aquatic systems, posing risks to ecosystems and human health . Traditional water treatment methods, such as ozonation and chlorination, are often ineffective for phenanthrene removal, necessitating advanced approaches like constructed wetlands (CWs), photocatalytic degradation, and microbial bioremediation .
Preparation Methods
Synthetic Routes and Reaction Conditions
One of the most common synthetic routes for phenanthrene is the Haworth phenanthrene synthesis. This multi-step process involves the following key steps :
Friedel-Crafts Acylation: Naphthalene is treated with succinic anhydride in the presence of aluminum chloride to form naphthoylpropionic acid.
Clemmensen Reduction: Naphthoylpropionic acid is reduced with amalgamated zinc in hydrochloric acid to give naphthobutyric acid.
Cyclization: The naphthobutyric acid undergoes cyclization to form phenanthrene.
Industrial Production Methods
Industrial production of phenanthrene typically involves the extraction from coal tar, a by-product of coal processing. The coal tar is distilled, and phenanthrene is separated from other PAHs through fractional distillation and recrystallization processes .
Chemical Reactions Analysis
Phenanthrene undergoes various chemical reactions, primarily at the 9 and 10 positions :
Reduction: Reduction of phenanthrene with hydrogen gas and Raney nickel yields 9,10-dihydrophenanthrene.
Halogenation: Electrophilic halogenation with bromine produces 9-bromophenanthrene.
Sulfonation: Aromatic sulfonation with sulfuric acid forms 2- and 3-phenanthrenesulfonic acids.
Ozonolysis: Ozonolysis of phenanthrene results in the formation of diphenylaldehyde.
Scientific Research Applications
Detection Methods
Electrochemical Immunosensors
Recent advancements have led to the development of electrochemical immunosensors for detecting phenanthrene in marine environments. A study utilized a multi-walled carbon nanotube-chitosan oligosaccharide nanocomposite membrane loaded with phenanthrene antibodies. This sensor demonstrated a high recovery rate (96.1% to 101.5%) and a detection limit of 0.30 ng/mL, making it a promising tool for real-time monitoring of phenanthrene in seawater .
Spectroscopic Studies
Phenanthrene's interactions with water have been characterized using rotational spectroscopy, revealing noncovalent interactions that play significant roles in chemical and biological systems. The study highlighted how these interactions can influence the behavior of phenanthrene in aqueous environments, which is crucial for understanding its fate and transport in natural waters .
Toxicity Assessments
Acute Toxicity Studies
Phenanthrene is known for its toxicity to aquatic organisms. Research has shown that the toxicity increases significantly with decreasing pH levels in seawater. For example, the effective concentration (EC50) required to inhibit algal growth decreased from 1.893 mg/L at pH 9.0 to 0.237 mg/L at pH 6.0, indicating that acidification enhances the toxic effects of phenanthrene on marine life .
Chronic Toxicity Criteria Development
A comprehensive study developed water quality criteria for phenanthrene based on acute and chronic toxicity tests across various aquatic species. The derived maximum concentration was set at 0.0514 mg/L, while the continuous concentration was 0.0186 mg/L, providing essential guidelines for assessing ecological risks associated with phenanthrene exposure in aquatic environments .
Biodegradation Studies
Soil Microbial Activity
Research into the biodegradation of phenanthrene has revealed that soil water content significantly affects its mineralization rates. A study indicated that optimal moisture levels enhance microbial degradation processes, with findings showing that up to 0.23% of phenanthrene was mineralized to carbon dioxide over a 66-day incubation period . This underscores the importance of environmental conditions in managing phenanthrene contamination.
Bioaccumulation and Ecological Impact
Bioaccumulation Studies
Phenanthrene exhibits notable bioaccumulation potential in aquatic organisms. Studies have demonstrated that amphipods exposed to water-only conditions accumulated significantly higher concentrations of phenanthrene compared to those exposed through sediment uptake. This finding emphasizes the need for monitoring water quality to prevent toxic accumulations in aquatic food webs .
Mechanism of Action
Phenanthrene exerts its effects through various molecular mechanisms. It is known to interact with cellular components, leading to oxidative stress and DNA damage. The compound’s carcinogenic properties are attributed to its ability to form reactive metabolites that bind to DNA, causing mutations and potentially leading to cancer . Phenanthrene also affects cellular signaling pathways, contributing to its toxic effects .
Comparison with Similar Compounds
Structural and Physicochemical Properties
Phenanthrene shares structural similarities with other PAHs but exhibits distinct physicochemical traits:
Compound | Structure | Water Solubility (mg/L) | Log Kow | Persistence |
---|---|---|---|---|
Phenanthrene | 3 fused benzene rings | 1.1 | 4.5 | High (weeks to months) |
Anthracene | Linear 3-ring system | 0.045 | 4.5 | Moderate |
Naphthalene | 2 fused benzene rings | 31.7 | 3.3 | Low (days to weeks) |
Pyrene | 4 fused benzene rings | 0.135 | 5.2 | Very high |
Key Differences :
- Hydrophobicity : Pyrene’s higher log Kow increases adsorption to sediments, whereas phenanthrene’s intermediate hydrophobicity allows broader dispersion .
- Detection Challenges : Anthracene and phenanthrene have overlapping chromatographic peaks due to similar retention times, complicating analytical differentiation .
Degradation Pathways and Efficiency
Microbial Degradation
- Phenanthrene : Degraded via both ortho- and meta-cleavage pathways by bacteria like Arthrobacter phenanivorans, producing phthalate intermediates . Co-metabolism with acenaphthene or fluorene enhances degradation rates (up to 93% in FTWs with bacterial consortia) .
- Anthracene : Requires specialized dioxygenases for initial hydroxylation, with slower degradation rates due to its linear structure .
Photocatalytic and Adsorptive Removal
- Phenanthrene’s floc structure and oxygen-containing functional groups make it highly adsorbable to graphene-coated materials (99% removal efficiency) . In contrast, naphthalene’s volatility reduces adsorption efficacy .
- Photocatalysis using TiO₂-based nanocomposites achieves 85–95% phenanthrene degradation, outperforming ozonation (~50% efficiency) .
Environmental Behavior and Interactions
- Temperature Effects : Phenanthrene solubility decreases with rising water temperatures, increasing partitioning into organic matter .
- Co-Contaminant Dynamics : In CWs, phenanthrene removal (81–93% COD reduction) is enhanced by plant-bacterial synergisms (Phragmites australis supports rhizosphere microbes) . However, heavy metals (e.g., Pb/Cd) can inhibit degradation by binding to PAH-degrading enzymes .
- Dissolved Oxygen (DO) : Phenanthrene shows a positive correlation with DO, unlike fluoranthene or benzo(a)pyrene, which degrade faster under anaerobic conditions .
Detection and Monitoring
- Fluorescence Spectroscopy : Phenanthrene exhibits unique excitation/emission peaks (λex = 250 nm, λem = 365 nm), distinguishable from anthracene (λem = 400 nm) .
- Synchronous Fluorescence: At Δλ = 100 nm, phenanthrene and naphthalene can be simultaneously quantified with detection limits <0.03 μg/L .
Ecological and Health Risks
Phenanthrene is classified as a Group 3 carcinogen (IARC), with toxicity mediated through DNA adduct formation. Its ecological risk quotient (RQ) in water is lower than benzo(a)pyrene (RQ > 1) but higher than naphthalene .
Biological Activity
Phenanthrene, a polycyclic aromatic hydrocarbon (PAH), is widely recognized for its environmental persistence and toxicity. Its biological activity, particularly in aquatic environments, has significant implications for ecological health and bioremediation strategies. This article explores the biological activity of phenanthrene in water, focusing on its degradation by microbial consortia, bioaccumulation in aquatic organisms, and the development of detection methods.
1. Microbial Degradation of Phenanthrene
Microbial Consortia for Degradation
Recent studies have highlighted the role of specific bacterial consortia in the degradation of phenanthrene. For instance, a study conducted in Antarctic soils isolated three bacterial consortia (C13, C15, and C23) capable of degrading phenanthrene by 45% to 85% within five days at a concentration of 50 mg/L and a temperature of 15 °C. The dominant genera identified were Pseudomonas and Pseudarthrobacter, which exhibited significant degradation capabilities under varying environmental conditions, including temperature fluctuations from 4 °C to 30 °C and different water availabilities .
Table 1: Phenanthrene Degradation by Bacterial Consortia
Consortium | Degradation Percentage (%) | Temperature (°C) | Water Availability |
---|---|---|---|
C13 | 85 | 15 | Variable |
C15 | 60 | 15 | Variable |
C23 | 45 | 15 | Variable |
2. Bioaccumulation in Aquatic Organisms
Bioaccumulation Studies
Phenanthrene's bioaccumulation potential has been extensively studied, particularly in aquatic organisms like amphipods and fish. Research indicates that amphipods exposed to phenanthrene in water accumulated significantly higher concentrations compared to those exposed through sediment. For example, the bioaccumulation factor (BAF) was calculated to be as high as 1380.38 for certain species when exposed solely to water .
Table 2: Bioaccumulation Factors for Aquatic Organisms
Species | Bioaccumulation Factor (BAF) | Concentration (µmol/g) | Toxicity Effects |
---|---|---|---|
Diporeia spp. | Up to 24 times higher | 5.8 | Slight toxicity |
Daphnia magna | Log BCF of 2.51 | Not specified | Not toxic |
Oncorhynchus mykiss | Physiological effects | 30 mg/kg | Induction of hepatic enzymes |
3. Detection Methods for Phenanthrene
Electrochemical Immunosensors
Recent advancements in detection technologies have led to the development of electrochemical immunosensors that can accurately detect phenanthrene in marine environments. A study utilized a multi-walled carbon nanotube-chitosan oligosaccharide nanocomposite membrane loaded with phenanthrene antibodies. This method demonstrated a detection limit as low as 0.30 ng/mL and was effective in real-time monitoring of phenanthrene levels in seawater .
Case Studies
Case Study: Antarctic Soil Microbial Consortia
In a controlled experiment, microbial consortia from Antarctic soils were tested for their ability to degrade phenanthrene under various conditions. The results showed that consortium C13 not only had the highest degradation rate but also maintained activity across a range of temperatures and water contents, indicating its potential application in bioremediation efforts in extreme environments .
Case Study: Bioaccumulation in Amphipods
A comprehensive study on the bioaccumulation of phenanthrene by amphipods revealed that exposure to water resulted in significantly higher tissue concentrations compared to sediment exposure. The findings indicated that the concentration required to produce biological effects was much higher than previously predicted using equilibrium-partitioning approaches .
Q & A
Basic Research Questions
Q. What methodologies are recommended for detecting phenanthrene in water with high sensitivity and accuracy?
Fluorescence spectroscopy coupled with N-way partial least squares (N-PLS) analysis is a robust approach. Phenanthrene exhibits distinct fluorescence peaks at excitation/emission wavelengths of 285/245 nm and 315/345 nm. N-PLS optimizes detection by resolving spectral overlaps from co-existing polycyclic aromatic hydrocarbons (PAHs), achieving prediction errors below 0.4 μg·L⁻¹ and relative errors <6% for phenanthrene in aqueous solutions . Calibration with synthetic and real water samples improves model reliability.
Q. How do physicochemical factors influence phenanthrene solubility and bioavailability in water systems?
Phenanthrene’s hydrophobicity (log Kow ~4.5) limits aqueous solubility, but bioavailability is enhanced by dissolved organic matter (DOM) and surfactants, which increase partitioning into the aqueous phase. Experimental studies using passive dosing via polymer-loaded systems show that low concentrations (μg·L⁻¹ range) and temperature variations (10–25°C) significantly affect biodegradation rates, with half-lives ranging from 3.5 to 16 days .
Q. What experimental designs are effective for assessing phenanthrene bioavailability in sediment-water systems?
Column studies with sediment slurries and controlled bubble mixing simulate natural resuspension. Filter-based corrections (e.g., 0.7 µm glass fiber) account for phenanthrene sorption losses during sampling. Concurrent measurement of total suspended solids (TSS) and porewater concentrations via HPLC (EPA Method 8270) ensures accurate quantification .
Advanced Research Questions
Q. How can researchers resolve contradictions between biodegradation studies and regulatory persistence classifications for phenanthrene?
A weight-of-evidence approach integrating ready biodegradability tests (e.g., OECD 301) and simulation data is critical. While regulatory assessments may cite sediment persistence, phenanthrene’s rapid degradation in water (half-life geomean: 3.5 days) and soil (OECD 307) supports its non-persistence classification. Discrepancies arise from compartment-specific thresholds; cross-referencing standardized tests with field data (e.g., Anacostia River flux models) clarifies environmental fate .
Q. What transport models best predict phenanthrene flux from sediment to water columns?
Finite-layer diffusion models (e.g., RECOVERY 4.3.1) simulate phenanthrene release under lab- and field-scale conditions. These models assume uniform initial sediment concentrations and zero basal flux. Experimental validation shows linear flux increases over time, aligning with first-order kinetics. Discrepancies between model outputs and field data require adjustments for bioirrigation and resuspension effects .
Q. What mechanisms explain the biphasic bioaccumulation of phenanthrene in benthic organisms?
Bioaccumulation in organisms like Lumbriculus variegatus involves rapid absorption from porewater (initial 12-hour phase) followed by slower ingestion of sediment-bound phenanthrene. Tissue concentration drops (e.g., 12–24 hours) may reflect metabolic transformation or excretion, though mechanistic studies are needed. Steady-state concentrations correlate with sediment organic carbon content .
Q. How can researchers optimize biodegradation studies to account for co-substrate effects in phenanthrene-spiked water?
Use passive dosing systems (e.g., silicone O-rings) to maintain stable aqueous concentrations without solvent interference. Co-metabolism experiments with acetate or glucose as co-substrates enhance microbial activity. Temperature-controlled incubations (20–25°C) and inocula pre-adapted to PAHs improve degradation rates .
Q. What statistical approaches address methodological limitations in phenanthrene detection?
Bilinear decomposition corrects concentration residuals in fluorescence datasets, reducing prediction errors for multi-component systems. Iterative N-PLS parameter optimization (e.g., iteration counts with minimal RMS error) enhances accuracy in heterogeneous water matrices .
Properties
CAS No. |
919080-09-4 |
---|---|
Molecular Formula |
C14H12O |
Molecular Weight |
196.24 g/mol |
IUPAC Name |
phenanthrene;hydrate |
InChI |
InChI=1S/C14H10.H2O/c1-3-7-13-11(5-1)9-10-12-6-2-4-8-14(12)13;/h1-10H;1H2 |
InChI Key |
JPKIKAADNMFDRS-UHFFFAOYSA-N |
Canonical SMILES |
C1=CC=C2C(=C1)C=CC3=CC=CC=C32.O |
Origin of Product |
United States |
Disclaimer and Information on In-Vitro Research Products
Please be aware that all articles and product information presented on BenchChem are intended solely for informational purposes. The products available for purchase on BenchChem are specifically designed for in-vitro studies, which are conducted outside of living organisms. In-vitro studies, derived from the Latin term "in glass," involve experiments performed in controlled laboratory settings using cells or tissues. It is important to note that these products are not categorized as medicines or drugs, and they have not received approval from the FDA for the prevention, treatment, or cure of any medical condition, ailment, or disease. We must emphasize that any form of bodily introduction of these products into humans or animals is strictly prohibited by law. It is essential to adhere to these guidelines to ensure compliance with legal and ethical standards in research and experimentation.