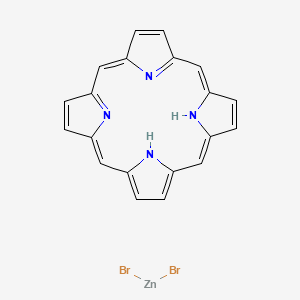
Dibromo zinc porphyrin
- Click on QUICK INQUIRY to receive a quote from our team of experts.
- With the quality product at a COMPETITIVE price, you can focus more on your research.
Overview
Description
Dibromo zinc porphyrin is a metalloporphyrin compound where a zinc ion is coordinated to a porphyrin ring that has two bromine atoms attached. Porphyrins are a class of organic compounds that play a crucial role in various biological systems, such as hemoglobin and chlorophyll. The incorporation of zinc and bromine into the porphyrin structure imparts unique chemical and physical properties to the compound, making it valuable for various scientific and industrial applications.
Preparation Methods
Synthetic Routes and Reaction Conditions
The synthesis of dibromo zinc porphyrin typically involves the complexation of dibromo porphyrins with zinc acetate. The process begins with the preparation of dibromo porphyrins, which can be achieved through the bromination of porphyrins using bromine or N-bromosuccinimide (NBS) as the brominating agents. The dibromo porphyrins are then reacted with zinc acetate in a suitable solvent, such as methanol or chloroform, under reflux conditions to form this compound .
Industrial Production Methods
Industrial production of this compound follows similar synthetic routes but on a larger scale. The process involves the use of industrial-grade reagents and solvents, and the reactions are carried out in large reactors with precise control over temperature, pressure, and reaction time to ensure high yield and purity of the final product .
Chemical Reactions Analysis
Types of Reactions
Dibromo zinc porphyrin undergoes various chemical reactions, including:
Substitution Reactions: The bromine atoms in this compound can be substituted with other functional groups through nucleophilic substitution reactions.
Oxidation and Reduction Reactions: The porphyrin ring can undergo oxidation and reduction reactions, which can alter the electronic properties of the compound.
Complexation Reactions: This compound can form complexes with various ligands, such as phosphines and amines, through coordination to the zinc ion.
Common Reagents and Conditions
Nucleophilic Substitution: Alkoxides, amines, thiols; solvents like methanol, ethanol; reaction conditionsroom temperature to reflux.
Oxidation: Hydrogen peroxide, oxygen; solvents like acetonitrile, dichloromethane; reaction conditionsroom temperature to elevated temperatures.
Major Products Formed
Substitution Products: Alkoxy, amino, or thio-substituted zinc porphyrins.
Oxidation Products: Oxidized porphyrin derivatives with altered electronic properties.
Reduction Products: Reduced porphyrin derivatives with modified conjugation.
Scientific Research Applications
Dibromo zinc porphyrin has a wide range of applications in scientific research:
Mechanism of Action
The mechanism of action of dibromo zinc porphyrin involves its ability to interact with various molecular targets through coordination to the zinc ion and the porphyrin ring. In photodynamic therapy, for example, this compound acts as a photosensitizer that, upon light irradiation, generates reactive oxygen species (ROS) such as singlet oxygen. These ROS cause oxidative damage to tumor cells, leading to cell death . The compound’s antimicrobial activity is attributed to its ability to disrupt microbial cell membranes and interfere with essential cellular processes .
Comparison with Similar Compounds
Similar Compounds
Dibromo magnesium porphyrin: Similar structure but with magnesium instead of zinc. Exhibits different electronic properties and reactivity.
Dibromo copper porphyrin: Contains copper instead of zinc. Known for its catalytic activity in redox reactions.
Uniqueness
Dibromo zinc porphyrin is unique due to its specific electronic and photophysical properties imparted by the zinc ion. It exhibits high thermal stability, strong absorption in the visible region, and efficient generation of reactive oxygen species, making it particularly valuable for applications in photodynamic therapy and advanced material development .
Properties
Molecular Formula |
C20H14Br2N4Zn |
---|---|
Molecular Weight |
535.5 g/mol |
IUPAC Name |
dibromozinc;21,22-dihydroporphyrin |
InChI |
InChI=1S/C20H14N4.2BrH.Zn/c1-2-14-10-16-5-6-18(23-16)12-20-8-7-19(24-20)11-17-4-3-15(22-17)9-13(1)21-14;;;/h1-12,21-22H;2*1H;/q;;;+2/p-2 |
InChI Key |
LJJDIPCSZWDPAR-UHFFFAOYSA-L |
Canonical SMILES |
C1=CC2=CC3=CC=C(N3)C=C4C=CC(=N4)C=C5C=CC(=N5)C=C1N2.[Zn](Br)Br |
Origin of Product |
United States |
Disclaimer and Information on In-Vitro Research Products
Please be aware that all articles and product information presented on BenchChem are intended solely for informational purposes. The products available for purchase on BenchChem are specifically designed for in-vitro studies, which are conducted outside of living organisms. In-vitro studies, derived from the Latin term "in glass," involve experiments performed in controlled laboratory settings using cells or tissues. It is important to note that these products are not categorized as medicines or drugs, and they have not received approval from the FDA for the prevention, treatment, or cure of any medical condition, ailment, or disease. We must emphasize that any form of bodily introduction of these products into humans or animals is strictly prohibited by law. It is essential to adhere to these guidelines to ensure compliance with legal and ethical standards in research and experimentation.