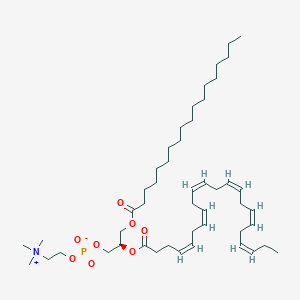
1-octadecanoyl-2-(4Z,7Z,10Z,13Z,16Z,19Z-docosahexaenoyl)-sn-glycero-3-phosphocholine
Overview
Description
1-Octadecanoyl-2-(4Z,7Z,10Z,13Z,16Z,19Z-docosahexaenoyl)-sn-glycero-3-phosphocholine is a 1,2-diacyl-sn-glycero-3-phosphoethanolamine in which the acyl substituents at positions 1 and 2 are specified as octadecanoyl and (4Z,7Z,10Z,13Z,16Z,19Z)-docosahexaenoyl respectively .
Physical And Chemical Properties Analysis
The molecular formula of this compound is C45H78NO8P. It has an average mass of 792.076 Da and a monoisotopic mass of 791.546509 Da . Another related compound, 1-octadecanoyl-2-(4Z,7Z,10Z,13Z,16Z,19Z-docosahexaenoyl)-sn-glycero-3-phosphoserine, has a molecular formula of C46H78NO10P, an average mass of 836.1 g/mol, and a monoisotopic mass of 835.536316 Da .Scientific Research Applications
Neurodegenerative Disease Research
This compound has been utilized in the study of lipid membrane organization and dynamics, which are crucial in understanding neurodegenerative diseases like Amyotrophic Lateral Sclerosis (ALS) . Alterations in the levels of this phospholipid have been observed in the L5 anterior horn in a transgenic mouse model of ALS, particularly at the terminal stage of the disease .
Liposome Preparation
In pharmaceutical research, liposomes are often used for drug delivery. This phospholipid has been employed in the preparation of liposomes to study the effects of fatty acid profiles on membrane vesiculation by proteins such as dynamin and endophilin .
Anti-Angiogenic Properties
Research has indicated that 1-stearoyl-2-docosahexaenoyl-sn-glycero-3-phosphocholine exhibits anti-angiogenic effects, which is the process of forming new blood vessels. It significantly reduces the proliferation, migration, and tube formation of human umbilical vein endothelial cells, suggesting potential in anti-tumor angiogenesis studies .
Miscibility Studies
This phospholipid has been used in miscibility studies to understand how different lipid species interact within the membrane. Such studies are essential for comprehending the heterogeneity and domain formation in biological membranes .
properties
IUPAC Name |
[(2R)-2-[(4Z,7Z,10Z,13Z,16Z,19Z)-docosa-4,7,10,13,16,19-hexaenoyl]oxy-3-octadecanoyloxypropyl] 2-(trimethylazaniumyl)ethyl phosphate | |
---|---|---|
Source | PubChem | |
URL | https://pubchem.ncbi.nlm.nih.gov | |
Description | Data deposited in or computed by PubChem | |
InChI |
InChI=1S/C48H84NO8P/c1-6-8-10-12-14-16-18-20-22-23-24-25-27-29-31-33-35-37-39-41-48(51)57-46(45-56-58(52,53)55-43-42-49(3,4)5)44-54-47(50)40-38-36-34-32-30-28-26-21-19-17-15-13-11-9-7-2/h8,10,14,16,20,22,24-25,29,31,35,37,46H,6-7,9,11-13,15,17-19,21,23,26-28,30,32-34,36,38-45H2,1-5H3/b10-8-,16-14-,22-20-,25-24-,31-29-,37-35-/t46-/m1/s1 | |
Source | PubChem | |
URL | https://pubchem.ncbi.nlm.nih.gov | |
Description | Data deposited in or computed by PubChem | |
InChI Key |
FAUYAENFVCNTAL-PFFNLMTBSA-N | |
Source | PubChem | |
URL | https://pubchem.ncbi.nlm.nih.gov | |
Description | Data deposited in or computed by PubChem | |
Canonical SMILES |
CCCCCCCCCCCCCCCCCC(=O)OCC(COP(=O)([O-])OCC[N+](C)(C)C)OC(=O)CCC=CCC=CCC=CCC=CCC=CCC=CCC | |
Source | PubChem | |
URL | https://pubchem.ncbi.nlm.nih.gov | |
Description | Data deposited in or computed by PubChem | |
Isomeric SMILES |
CCCCCCCCCCCCCCCCCC(=O)OC[C@H](COP(=O)([O-])OCC[N+](C)(C)C)OC(=O)CC/C=C\C/C=C\C/C=C\C/C=C\C/C=C\C/C=C\CC | |
Source | PubChem | |
URL | https://pubchem.ncbi.nlm.nih.gov | |
Description | Data deposited in or computed by PubChem | |
Molecular Formula |
C48H84NO8P | |
Source | PubChem | |
URL | https://pubchem.ncbi.nlm.nih.gov | |
Description | Data deposited in or computed by PubChem | |
DSSTOX Substance ID |
DTXSID301335920 | |
Record name | 1-Stearoyl-2-docosahexaenoyl-sn-glycero-3-phosphocholine | |
Source | EPA DSSTox | |
URL | https://comptox.epa.gov/dashboard/DTXSID301335920 | |
Description | DSSTox provides a high quality public chemistry resource for supporting improved predictive toxicology. | |
Molecular Weight |
834.2 g/mol | |
Source | PubChem | |
URL | https://pubchem.ncbi.nlm.nih.gov | |
Description | Data deposited in or computed by PubChem | |
Physical Description |
Solid | |
Record name | PC(18:0/22:6(4Z,7Z,10Z,13Z,16Z,19Z)) | |
Source | Human Metabolome Database (HMDB) | |
URL | http://www.hmdb.ca/metabolites/HMDB0008057 | |
Description | The Human Metabolome Database (HMDB) is a freely available electronic database containing detailed information about small molecule metabolites found in the human body. | |
Explanation | HMDB is offered to the public as a freely available resource. Use and re-distribution of the data, in whole or in part, for commercial purposes requires explicit permission of the authors and explicit acknowledgment of the source material (HMDB) and the original publication (see the HMDB citing page). We ask that users who download significant portions of the database cite the HMDB paper in any resulting publications. | |
Product Name |
1-octadecanoyl-2-(4Z,7Z,10Z,13Z,16Z,19Z-docosahexaenoyl)-sn-glycero-3-phosphocholine | |
CAS RN |
59403-52-0 | |
Record name | 1-Stearoyl-2-docosahexaenoyl-sn-glycero-3-phosphocholine | |
Source | EPA DSSTox | |
URL | https://comptox.epa.gov/dashboard/DTXSID301335920 | |
Description | DSSTox provides a high quality public chemistry resource for supporting improved predictive toxicology. | |
Retrosynthesis Analysis
AI-Powered Synthesis Planning: Our tool employs the Template_relevance Pistachio, Template_relevance Bkms_metabolic, Template_relevance Pistachio_ringbreaker, Template_relevance Reaxys, Template_relevance Reaxys_biocatalysis model, leveraging a vast database of chemical reactions to predict feasible synthetic routes.
One-Step Synthesis Focus: Specifically designed for one-step synthesis, it provides concise and direct routes for your target compounds, streamlining the synthesis process.
Accurate Predictions: Utilizing the extensive PISTACHIO, BKMS_METABOLIC, PISTACHIO_RINGBREAKER, REAXYS, REAXYS_BIOCATALYSIS database, our tool offers high-accuracy predictions, reflecting the latest in chemical research and data.
Strategy Settings
Precursor scoring | Relevance Heuristic |
---|---|
Min. plausibility | 0.01 |
Model | Template_relevance |
Template Set | Pistachio/Bkms_metabolic/Pistachio_ringbreaker/Reaxys/Reaxys_biocatalysis |
Top-N result to add to graph | 6 |
Feasible Synthetic Routes
Q & A
A: SDPC possesses a saturated stearoyl chain (18:0) at the sn-1 position and a highly unsaturated docosahexaenoyl chain (22:6ω3) at the sn-2 position, unlike POPC, which has a monounsaturated oleoyl chain (18:1) at the sn-2 position. This difference in unsaturation significantly impacts its behavior in bilayers. Neutron diffraction studies reveal that the polyunsaturated docosahexaenoyl chain prefers to reside near the water interface, leaving the saturated stearoyl chain to fill the voids in the bilayer center []. This uneven chain distribution creates membrane elastic stress potentially influencing the function of embedded proteins like rhodopsin. Furthermore, SDPC bilayers, despite having longer acyl chains than POPC, exhibit similar hydrophobic thicknesses in the fluid phase due to the extended conformation of polyunsaturated chains in the gel phase and their unique packing properties in the fluid phase [].
A: Cholesterol exhibits complex interactions with SDPC in bilayers. Molecular dynamics simulations have demonstrated that cholesterol prefers to interact with the saturated stearoyl chains of SDPC rather than the polyunsaturated docosahexaenoyl chains []. This preferential interaction can lead to the formation of laterally inhomogeneous membranes, potentially contributing to the formation of lipid rafts. Additionally, while cholesterol generally increases membrane order, in SDPC bilayers containing both cholesterol and the symmetric polyunsaturated lipid 1,2-docosahexaenoyl-sn-glycero-3-phosphocholine, cholesterol suppresses the formation of non-lamellar structures typically induced by the symmetric lipid. This leads to a bilayer with unique packing characteristics that cannot be classified as either liquid-ordered or liquid-disordered [].
A: SDPC plays a significant role in modulating GPCR signaling by influencing the kinetics of receptor-G protein complex formation. Studies using rhodopsin and transducin as a model system show that the rate of complex formation is significantly faster in SDPC bilayers compared to POPC bilayers, especially those containing cholesterol []. This suggests that the biophysical properties of SDPC, potentially related to its influence on membrane fluidity and lateral pressure profiles, facilitate efficient coupling between rhodopsin and transducin, thereby influencing the overall signaling response [].
A: Molecular dynamics simulations reveal distinct interactions of anesthetic molecules like halothane with SDPC bilayers compared to saturated bilayers []. While halothane primarily resides near the upper part of the acyl chains in both bilayer types, an additional density maximum is observed at the membrane center specifically in SDPC bilayers. This difference likely arises from the unique packing properties of SDPC due to its highly unsaturated docosahexaenoyl chains. Despite these differences, the impact of halothane on the electrostatic properties of the membrane interface remains similar between SDPC and saturated lipid bilayers, characterized by a shift in the orientation of lipid headgroup dipoles towards the membrane interior.
Disclaimer and Information on In-Vitro Research Products
Please be aware that all articles and product information presented on BenchChem are intended solely for informational purposes. The products available for purchase on BenchChem are specifically designed for in-vitro studies, which are conducted outside of living organisms. In-vitro studies, derived from the Latin term "in glass," involve experiments performed in controlled laboratory settings using cells or tissues. It is important to note that these products are not categorized as medicines or drugs, and they have not received approval from the FDA for the prevention, treatment, or cure of any medical condition, ailment, or disease. We must emphasize that any form of bodily introduction of these products into humans or animals is strictly prohibited by law. It is essential to adhere to these guidelines to ensure compliance with legal and ethical standards in research and experimentation.