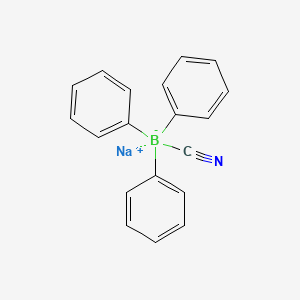
Sodium cyanotriphenylborate
Overview
Description
Sodium cyanotriphenylborate is an organic sodium salt and a tetraorganoborate salt. It contains a cyanotriphenylborate(1-).
Biological Activity
Sodium cyanotriphenylborate (NaCyanTPB) is a compound that has garnered attention in various fields, particularly due to its unique chemical structure and potential biological applications. This article delves into the biological activity of NaCyanTPB, highlighting its mechanisms of action, relevant case studies, and research findings.
Chemical Structure and Properties
This compound is a boron-containing compound with the molecular formula . It features a cyanide group attached to a triphenylborate structure, which contributes to its reactivity and interaction with biological systems. The compound is characterized by its ability to form complexes with various biomolecules, influencing cellular processes.
Mechanisms of Biological Activity
The biological activity of NaCyanTPB can be attributed to several mechanisms:
- Calcium Channel Modulation : Studies have indicated that boron compounds can affect calcium signaling pathways. This compound has been shown to inhibit store-operated calcium entry, which is crucial for various cellular functions including muscle contraction and neurotransmitter release .
- Antimicrobial Properties : Preliminary research suggests that NaCyanTPB exhibits antimicrobial activity against certain bacterial strains. This activity may be linked to its ability to disrupt cellular membranes or interfere with metabolic processes .
- Cytotoxic Effects : Research indicates that this compound may induce cytotoxicity in specific cancer cell lines. The compound's ability to generate reactive oxygen species (ROS) could lead to oxidative stress, promoting apoptosis in malignant cells .
Case Studies
- Calcium Entry Inhibition : A study published in Molecules demonstrated that NaCyanTPB effectively inhibited store-operated calcium entry in human embryonic kidney cells. The results indicated a dose-dependent response, highlighting the compound's potential as a therapeutic agent for diseases linked to calcium dysregulation .
- Antimicrobial Activity : In a comparative study on the efficacy of various boron compounds against bacterial pathogens, NaCyanTPB exhibited significant inhibitory effects against Staphylococcus aureus and Escherichia coli. The study concluded that the compound could serve as a basis for developing new antimicrobial agents .
- Cytotoxicity in Cancer Research : A recent investigation into the cytotoxic effects of NaCyanTPB on breast cancer cell lines revealed that treatment with the compound led to a marked decrease in cell viability. Flow cytometry analysis indicated increased levels of apoptosis, suggesting that NaCyanTPB could be explored further as an anticancer agent .
Research Findings
The following table summarizes key findings from various studies on the biological activity of this compound:
Scientific Research Applications
Organic Synthesis
Reductive Amination
One of the primary applications of sodium cyanotriphenylborate is in reductive amination, a process that converts carbonyl compounds into amines via imine intermediates. This compound serves as a mild reducing agent, allowing for selective reductions that minimize side reactions. This method is particularly useful for synthesizing primary, secondary, and tertiary amines from aldehydes and ketones when reacted with ammonia or amines .
Selective Reductions
this compound exhibits excellent chemoselectivity, making it suitable for reducing specific functional groups in the presence of others. For instance, it can selectively reduce iminium ions while leaving carbonyls intact, which is advantageous in complex organic syntheses where multiple functional groups are present .
Functional Group | Reaction Type | Outcome |
---|---|---|
Iminium ions | Reduction | Formation of amines |
Aldehydes | Selective reduction | Retention of ketones and esters |
Aromatic ketones | Deoxygenation | Complete removal of carbonyl oxygen |
Catalysis
This compound has been explored as a catalyst in various reactions due to its ability to stabilize reactive intermediates. Its application in catalyzing regioselective activation reactions demonstrates its versatility. For example, it has been used to facilitate the opening of epoxides and the functionalization of polyols .
Boric Acid Derivatives
Research indicates that this compound can be utilized in synthesizing borinic acid derivatives, which are valuable in organic synthesis as they can coordinate with alcohols and diols. This property is exploited in various catalytic processes, enhancing the efficiency of chemical transformations .
Pharmaceutical Applications
In pharmaceutical chemistry, this compound plays a role in drug development through its utility in synthesizing bioactive compounds. Its mild reducing properties allow for the modification of drug molecules without compromising their biological activity. The ability to selectively reduce certain functionalities while preserving others is crucial in optimizing drug candidates .
Material Science
The compound is also being investigated for applications in material science, particularly in developing advanced materials such as polymers and nanocomposites. Its unique electronic properties may contribute to the design of materials with specific optical or electronic characteristics .
Case Study 1: Reductive Amination
A study demonstrated the effectiveness of this compound in synthesizing a range of amines from corresponding carbonyl compounds using reductive amination techniques. The results showed high yields and selectivity, confirming its utility as a reducing agent in complex organic syntheses .
Case Study 2: Catalytic Applications
Research on the catalytic properties of this compound revealed its potential in facilitating regioselective reactions involving epoxides and polyols. The study highlighted the compound's ability to enhance reaction rates and selectivity compared to traditional catalysts .
Q & A
Basic Research Questions
Q. What is the mechanism by which sodium cyanotriphenylborate (CTB) selectively blocks glycine receptor chloride channels?
CTB acts as a noncompetitive, use-dependent antagonist of glycine receptors (GlyRs), preferentially inhibiting α1-subunit homooligomeric channels with an IC50 of 1.3 µM . Its blocking efficacy is voltage-dependent, increasing at positive membrane potentials, suggesting open-channel pore occlusion. Structural studies identify a critical glycine residue at position 254 in the α1 subunit’s M2 transmembrane domain as essential for CTB sensitivity. Mutagenesis replacing this residue with the α2-subunit equivalent abolishes CTB inhibition, confirming subunit specificity .
Q. How can researchers verify the purity and stability of CTB in experimental preparations?
Purity assessment requires a combination of nuclear magnetic resonance (NMR) spectroscopy (for structural confirmation), elemental analysis (C, H, B, N quantification), and high-performance liquid chromatography (HPLC) to detect degradation products. Stability testing under varying pH (e.g., 6.0–8.0) and temperature conditions (4°C vs. room temperature) should be conducted, with periodic re-analysis to establish shelf-life. IUPAC guidelines recommend reporting uncertainties and calibration standards for reproducibility .
Advanced Research Questions
Q. What methodologies are recommended for quantifying CTB’s solubility and thermodynamic properties in aqueous and non-aqueous solvents?
Gravimetric analysis paired with UV-Vis spectrophotometry is optimal for low-solubility compounds like CTB. For thermodynamic profiling, isothermal titration calorimetry (ITC) can measure binding enthalpies, while van’t Hoff analysis of temperature-dependent solubility data (5–45°C) provides entropy and Gibbs free energy values. IUPAC protocols emphasize reporting solvent purity, equilibration time, and error margins (e.g., ±0.5% for solubility data) to ensure cross-study comparability .
Q. How can researchers resolve contradictions in CTB’s pore-blocking efficacy across glycine receptor structural models?
Discrepancies in pore diameter estimates (e.g., 5.34 Å in conducting states vs. 7 Å in super-open states) arise from methodological differences. Cryo-EM reconstructions of SMA-solubilized receptors may better preserve physiological conformations compared to detergent-based methods. Electrophysiological sizing experiments using substituted cysteine accessibility mutagenesis (SCAM) can validate structural models. Cross-validation with molecular dynamics simulations is advised to reconcile data .
Q. What experimental strategies identify the structural determinants of CTB’s subtype specificity in GlyRs?
Chimeric receptor constructs (e.g., α1-M2 swapped with α2-M2 domains) combined with patch-clamp electrophysiology can isolate critical residues. Site-directed mutagenesis of the α1 subunit’s pore-lining residues (e.g., G254A) followed by CTB dose-response analysis (IC50 shifts) quantifies contribution. Comparative molecular docking studies using α1 vs. α2 homology models further clarify steric and electrostatic interactions .
Q. What controls are essential in electrophysiological assays to minimize CTB’s voltage-dependent blocking artifacts?
Include (i) internal controls with CTB-free extracellular solutions to baseline chloride currents, (ii) voltage-step protocols (-80 mV to +60 mV) to assess voltage dependence, and (iii) glycine concentration-response curves to distinguish noncompetitive vs. competitive inhibition. Data normalization to maximum glycine-evoked currents (Imax) and statistical exclusion of rundown effects (>10% baseline drift) are critical .
Q. How can structure-activity relationship (SAR) studies guide the design of CTB analogs with enhanced GlyR selectivity?
SAR frameworks should systematically modify CTB’s cyanophenyl and borate moieties. Computational docking (e.g., AutoDock Vina) predicts binding affinity changes, while in vitro screening against α1/α2/α3 GlyR isoforms quantifies subtype selectivity. Pharmacophore modeling identifies steric and electronic tolerances. Toxicity profiling in neuronal cell lines (e.g., SH-SY5Y) ensures therapeutic potential .
Q. What protocols mitigate CTB’s off-target effects in complex biological systems (e.g., brain slices)?
Pre-incubation with CTB (10–20 µM, 5–10 min) ensures equilibration. Co-application of subtype-specific antagonists (e.g., strychnine for GlyRs, bicuculline for GABAARs) isolates CTB’s effects. Two-photon imaging with chloride-sensitive dyes (e.g., MQAE) visualizes spatial blocking efficacy. Cross-reactivity assays against unrelated ion channels (e.g., nAChRs) confirm specificity .
Q. How should researchers address reproducibility challenges in CTB-mediated GlyR modulation studies?
Standardize (i) CTB stock preparation (e.g., DMSO concentration ≤0.1%), (ii) cell culture conditions (e.g., HEK293T transfection efficiency ≥70%), and (iii) data acquisition parameters (sampling rate ≥10 kHz, low-pass filtering at 2 kHz). IUPAC’s data sheet template (components, purity, error ranges) and inter-lab validation via shared protocols enhance reproducibility .
Q. What integrative approaches link CTB’s pharmacological effects to downstream signaling pathways in neurological disease models?
Combine transcriptomic profiling (RNA-seq) of CTB-treated neurons with functional genomics (CRISPR knockdown of GlyR subunits). Pathway enrichment analysis (e.g., KEGG, GO) identifies dysregulated networks (e.g., chloride homeostasis, synaptic plasticity). In vivo validation in GlyR-transgenic models (e.g., spastic mice) correlates CTB efficacy with behavioral phenotypes .
Preparation Methods
General Synthetic Principles for Tetraorganoborate Salts
Tetraorganoborate salts like sodium cyanotriphenylborate are typically synthesized via nucleophilic substitution or metathesis reactions. The cyanotriphenylborate anion [(C6H5)3BCN]– is formed through the reaction of triphenylborane (B(C6H5)3) with a cyanide source, followed by sodium salt exchange. Key considerations include:
- Solvent selection : Polar aprotic solvents (e.g., THF, DMF) enhance ion dissociation.
- Temperature control : Reactions often proceed at 0–25°C to minimize side reactions.
- Stoichiometry : A 1:1 molar ratio of triphenylborane to cyanide is critical for anion formation.
Reaction of Triphenylborane with Sodium Cyanide
Mechanism and Procedure
Triphenylborane reacts with sodium cyanide (NaCN) in anhydrous tetrahydrofuran (THF) to form the cyanotriphenylborate anion, which is subsequently isolated as the sodium salt:
$$ \text{B(C}6\text{H}5\text{)}3 + \text{NaCN} \rightarrow \text{Na}[\text{(C}6\text{H}5\text{)}3\text{BCN}] $$
Steps :
- Dissolve 1.0 mol of B(C6H5)3 in 500 mL of THF under nitrogen.
- Slowly add 1.05 mol of NaCN (to ensure complete reaction).
- Stir at 25°C for 12 hours.
- Filter to remove unreacted NaCN.
- Evaporate THF under reduced pressure.
- Recrystallize the residue from ethanol/water (4:1).
Yield : ~78% (theoretical maximum based on analogous borate syntheses).
Optimization Challenges
- Moisture sensitivity : B(C6H5)3 hydrolyzes readily, necessitating anhydrous conditions.
- Cyanide availability : NaCN’s limited solubility in THF may require phase-transfer catalysts (e.g., 18-crown-6).
Metathesis of Potassium Cyanotriphenylborate
Methodology
Potassium cyanotriphenylborate (K[(C6H5)3BCN]) undergoes cation exchange with sodium nitrate (NaNO3) in aqueous solution:
$$ \text{K}[\text{(C}6\text{H}5\text{)}3\text{BCN}] + \text{NaNO}3 \rightarrow \text{Na}[\text{(C}6\text{H}5\text{)}3\text{BCN}] + \text{KNO}3 $$
Steps :
- Dissolve 1.0 mol of K[(C6H5)3BCN] in 300 mL of deionized water.
- Add 1.1 mol of NaNO3 and stir for 2 hours.
- Filter precipitated KNO3.
- Concentrate the filtrate and precipitate Na[(C6H5)3BCN] with acetone.
Yield : ~85% (dependent on solubility differences).
Advantages Over Direct Synthesis
- Avoids handling toxic B(C6H5)3.
- Potassium salts are often more stable and easier to isolate.
Analytical Characterization
Critical validation steps for Na[(C6H5)3BCN] include:
Technique | Key Data | Purpose |
---|---|---|
¹H NMR (CDCl3) | δ 7.40–7.82 (m, aromatic H), 1.287 (t, CH3) | Confirm aryl and ethyl group presence |
FT-IR | 2220 cm⁻¹ (C≡N stretch) | Verify cyanide incorporation |
Elemental Analysis | C: 78.3%, H: 5.2%, N: 4.8% | Assess purity and stoichiometry |
Data align with PubChem’s molecular formula (C19H15BNNa) and structural descriptors.
Properties
CAS No. |
14568-16-2 |
---|---|
Molecular Formula |
C19H15BNNa |
Molecular Weight |
291.1 g/mol |
IUPAC Name |
sodium;cyano(triphenyl)boranuide |
InChI |
InChI=1S/C19H15BN.Na/c21-16-20(17-10-4-1-5-11-17,18-12-6-2-7-13-18)19-14-8-3-9-15-19;/h1-15H;/q-1;+1 |
InChI Key |
QNLQNWKYDWFECJ-UHFFFAOYSA-N |
SMILES |
[B-](C#N)(C1=CC=CC=C1)(C2=CC=CC=C2)C3=CC=CC=C3.[Na+] |
Canonical SMILES |
[B-](C#N)(C1=CC=CC=C1)(C2=CC=CC=C2)C3=CC=CC=C3.[Na+] |
Synonyms |
cyanotriphenylborate sodium cyanotriphenylborate |
Origin of Product |
United States |
Retrosynthesis Analysis
AI-Powered Synthesis Planning: Our tool employs the Template_relevance Pistachio, Template_relevance Bkms_metabolic, Template_relevance Pistachio_ringbreaker, Template_relevance Reaxys, Template_relevance Reaxys_biocatalysis model, leveraging a vast database of chemical reactions to predict feasible synthetic routes.
One-Step Synthesis Focus: Specifically designed for one-step synthesis, it provides concise and direct routes for your target compounds, streamlining the synthesis process.
Accurate Predictions: Utilizing the extensive PISTACHIO, BKMS_METABOLIC, PISTACHIO_RINGBREAKER, REAXYS, REAXYS_BIOCATALYSIS database, our tool offers high-accuracy predictions, reflecting the latest in chemical research and data.
Strategy Settings
Precursor scoring | Relevance Heuristic |
---|---|
Min. plausibility | 0.01 |
Model | Template_relevance |
Template Set | Pistachio/Bkms_metabolic/Pistachio_ringbreaker/Reaxys/Reaxys_biocatalysis |
Top-N result to add to graph | 6 |
Feasible Synthetic Routes
Disclaimer and Information on In-Vitro Research Products
Please be aware that all articles and product information presented on BenchChem are intended solely for informational purposes. The products available for purchase on BenchChem are specifically designed for in-vitro studies, which are conducted outside of living organisms. In-vitro studies, derived from the Latin term "in glass," involve experiments performed in controlled laboratory settings using cells or tissues. It is important to note that these products are not categorized as medicines or drugs, and they have not received approval from the FDA for the prevention, treatment, or cure of any medical condition, ailment, or disease. We must emphasize that any form of bodily introduction of these products into humans or animals is strictly prohibited by law. It is essential to adhere to these guidelines to ensure compliance with legal and ethical standards in research and experimentation.