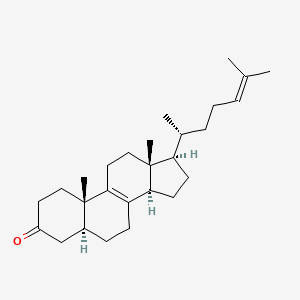
zymosterone
Overview
Description
Scientific Research Applications
Zymosterone is primarily used in research related to sterol biosynthesis. Its applications include:
Chemistry: Studying the metabolic pathways and enzymatic reactions involved in sterol biosynthesis.
Biology: Understanding the role of sterols in cellular processes and membrane structure.
Medicine: Investigating the potential therapeutic targets within the sterol biosynthesis pathway for treating diseases related to cholesterol metabolism.
Preparation Methods
Synthetic Routes and Reaction Conditions: Zymosterone can be synthesized through the metabolic engineering of yeast strains. One method involves using yeast mutants engineered in the ERG27 gene, which encodes 3-ketosteroid reductase. This enzyme is crucial for converting this compound to zymosterol . The preparation involves:
- Incubation of cell homogenates of an ERG27-deletant strain with radioactive lanosterol.
- Incubation of growing cells of a strain expressing point-mutated 3-ketosteroid reductase with radioactive acetate .
Industrial Production Methods: Industrial production of this compound is not widely documented, but it can be inferred that large-scale production would involve similar metabolic engineering techniques, optimizing yeast strains for higher yields and using bioreactors for controlled fermentation processes.
Types of Reactions:
Reduction: this compound undergoes reduction to form zymosterol, catalyzed by 3-keto sterol reductase (ERG27).
Oxidation: It can be oxidized to form various intermediates in the sterol biosynthesis pathway.
Common Reagents and Conditions:
Oxidation: Specific oxidizing agents and conditions are used depending on the desired intermediate or end product.
Major Products:
Mechanism of Action
Zymosterone exerts its effects through its role as an intermediate in the sterol biosynthesis pathway. It is converted to zymosterol by the enzyme 3-keto sterol reductase (ERG27), which is a key step in the production of cholesterol and ergosterol . The molecular targets and pathways involved include the enzymes and intermediates of the sterol biosynthesis pathway.
Comparison with Similar Compounds
Zymosterol: The direct product of zymosterone reduction.
Lanosterol: A precursor in the sterol biosynthesis pathway that is converted to zymosterol.
4-Methylthis compound: Another intermediate in the sterol biosynthesis pathway.
Uniqueness: this compound is unique due to its specific role in the sterol biosynthesis pathway, acting as a crucial intermediate that bridges the conversion of lanosterol to zymosterol. Its presence and conversion are essential for the proper functioning of the sterol biosynthesis pathway, making it a valuable compound for research and potential therapeutic applications .
Properties
IUPAC Name |
(5S,10S,13R,14R,17R)-10,13-dimethyl-17-[(2R)-6-methylhept-5-en-2-yl]-1,2,4,5,6,7,11,12,14,15,16,17-dodecahydrocyclopenta[a]phenanthren-3-one | |
---|---|---|
Source | PubChem | |
URL | https://pubchem.ncbi.nlm.nih.gov | |
Description | Data deposited in or computed by PubChem | |
InChI |
InChI=1S/C27H42O/c1-18(2)7-6-8-19(3)23-11-12-24-22-10-9-20-17-21(28)13-15-26(20,4)25(22)14-16-27(23,24)5/h7,19-20,23-24H,6,8-17H2,1-5H3/t19-,20+,23-,24+,26+,27-/m1/s1 | |
Source | PubChem | |
URL | https://pubchem.ncbi.nlm.nih.gov | |
Description | Data deposited in or computed by PubChem | |
InChI Key |
AUNLIRXIJAVBNM-ZSBATXSLSA-N | |
Source | PubChem | |
URL | https://pubchem.ncbi.nlm.nih.gov | |
Description | Data deposited in or computed by PubChem | |
Canonical SMILES |
CC(CCC=C(C)C)C1CCC2C1(CCC3=C2CCC4C3(CCC(=O)C4)C)C | |
Source | PubChem | |
URL | https://pubchem.ncbi.nlm.nih.gov | |
Description | Data deposited in or computed by PubChem | |
Isomeric SMILES |
C[C@H](CCC=C(C)C)[C@H]1CC[C@@H]2[C@@]1(CCC3=C2CC[C@@H]4[C@@]3(CCC(=O)C4)C)C | |
Source | PubChem | |
URL | https://pubchem.ncbi.nlm.nih.gov | |
Description | Data deposited in or computed by PubChem | |
Molecular Formula |
C27H42O | |
Source | PubChem | |
URL | https://pubchem.ncbi.nlm.nih.gov | |
Description | Data deposited in or computed by PubChem | |
DSSTOX Substance ID |
DTXSID801344697 | |
Record name | 5alpha-Cholesta-8,24-dien-3-one | |
Source | EPA DSSTox | |
URL | https://comptox.epa.gov/dashboard/DTXSID801344697 | |
Description | DSSTox provides a high quality public chemistry resource for supporting improved predictive toxicology. | |
Molecular Weight |
382.6 g/mol | |
Source | PubChem | |
URL | https://pubchem.ncbi.nlm.nih.gov | |
Description | Data deposited in or computed by PubChem | |
CAS No. |
27192-37-6 | |
Record name | 5alpha-Cholesta-8,24-dien-3-one | |
Source | EPA DSSTox | |
URL | https://comptox.epa.gov/dashboard/DTXSID801344697 | |
Description | DSSTox provides a high quality public chemistry resource for supporting improved predictive toxicology. | |
Retrosynthesis Analysis
AI-Powered Synthesis Planning: Our tool employs the Template_relevance Pistachio, Template_relevance Bkms_metabolic, Template_relevance Pistachio_ringbreaker, Template_relevance Reaxys, Template_relevance Reaxys_biocatalysis model, leveraging a vast database of chemical reactions to predict feasible synthetic routes.
One-Step Synthesis Focus: Specifically designed for one-step synthesis, it provides concise and direct routes for your target compounds, streamlining the synthesis process.
Accurate Predictions: Utilizing the extensive PISTACHIO, BKMS_METABOLIC, PISTACHIO_RINGBREAKER, REAXYS, REAXYS_BIOCATALYSIS database, our tool offers high-accuracy predictions, reflecting the latest in chemical research and data.
Strategy Settings
Precursor scoring | Relevance Heuristic |
---|---|
Min. plausibility | 0.01 |
Model | Template_relevance |
Template Set | Pistachio/Bkms_metabolic/Pistachio_ringbreaker/Reaxys/Reaxys_biocatalysis |
Top-N result to add to graph | 6 |
Feasible Synthetic Routes
Q1: What is the role of zymosterone in cholesterol biosynthesis and what happens if this step is blocked?
A1: this compound is a key intermediate in the cholesterol biosynthesis pathway. It is converted to zymosterol by the enzyme Hydroxysteroid (17β) Dehydrogenase 7 (HSD17B7), which acts as a 3-ketosteroid reductase [, ]. This enzymatic step is crucial for the proper progression of cholesterol synthesis. Studies using HSD17B7 knockout mice (HSD17B7KO) have shown that the absence of this enzyme leads to a blockage in de novo cholesterol biosynthesis in mouse embryos [, ]. As a consequence, these embryos die by embryonic day 10.5, highlighting the essential role of this compound conversion in embryonic development [, ].
Q2: What are the consequences of HSD17B7 deficiency beyond disrupted cholesterol synthesis?
A2: Beyond its role in cholesterol synthesis, HSD17B7 deficiency has severe consequences for embryonic development. Studies have revealed that HSD17B7KO mice exhibit defects in neuroectodermal survival and cardiovascular differentiation [, ]. These embryos show impaired development of forebrain hemispheres, increased apoptosis in neuronal tissues, and morphological abnormalities in the cardiovascular system, including reduced vascular complexity and heart defects [, ]. These findings underscore the broader biological importance of this compound processing by HSD17B7 during embryonic development.
Q3: How is the expression of HSD17B7, the enzyme responsible for this compound conversion, regulated?
A3: The transcriptional regulation of HSD17B7, the enzyme that converts this compound to zymosterol, is complex and involves various transcription factors. Both human and murine HSD17B7 promoters, despite low sequence similarity, share similar transcription factor binding site contexts []. Key regulators include the vitamin D receptor/retinoid X receptor, sterol regulatory element-binding protein (SREBP), and hepatocyte nuclear factor 4 (HNF4) []. These factors, particularly SREBP and HNF4, are known to regulate the expression of other genes involved in cholesterol biosynthesis, further supporting the role of HSD17B7 in this pathway [].
Q4: Can you provide an example of how researchers study the intermediates in the cholesterol biosynthesis pathway?
A4: Researchers utilize genetic engineering techniques to investigate intermediates in the cholesterol biosynthesis pathway. For instance, by manipulating the ERG27 gene in yeast, which encodes the 3-ketosteroid reductase enzyme, scientists can study the accumulation of 4-methyl this compound and other 4-methyl intermediates []. This approach allows for a deeper understanding of the individual steps involved in the pathway and the consequences of disrupting specific enzymatic reactions.
Disclaimer and Information on In-Vitro Research Products
Please be aware that all articles and product information presented on BenchChem are intended solely for informational purposes. The products available for purchase on BenchChem are specifically designed for in-vitro studies, which are conducted outside of living organisms. In-vitro studies, derived from the Latin term "in glass," involve experiments performed in controlled laboratory settings using cells or tissues. It is important to note that these products are not categorized as medicines or drugs, and they have not received approval from the FDA for the prevention, treatment, or cure of any medical condition, ailment, or disease. We must emphasize that any form of bodily introduction of these products into humans or animals is strictly prohibited by law. It is essential to adhere to these guidelines to ensure compliance with legal and ethical standards in research and experimentation.