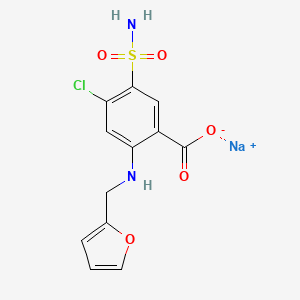
Sodium furosemide
Overview
Description
Furosemide sodium is a potent loop diuretic widely used in the medical field to treat conditions such as edema associated with heart failure, liver cirrhosis, and renal disease. It is also used to manage hypertension. Furosemide sodium works by inhibiting the reabsorption of sodium and chloride in the kidneys, leading to increased urine production and the removal of excess fluid from the body .
Mechanism of Action
Biochemical Analysis
Biochemical Properties
Sodium furosemide plays a crucial role in biochemical reactions by interacting with various enzymes, proteins, and other biomolecules. It primarily inhibits the sodium-potassium-chloride cotransporter (NKCC2) in the thick ascending limb of the loop of Henle . This inhibition prevents the reabsorption of sodium, chloride, and potassium, leading to their increased excretion in the urine . Additionally, this compound interacts with organic anion transporters (OAT1) in the proximal tubule, facilitating its secretion into the tubular lumen .
Cellular Effects
This compound exerts significant effects on various cell types and cellular processes. It disrupts the electrolyte balance within cells, leading to changes in cell volume and osmotic pressure . This disruption can influence cell signaling pathways, particularly those involving sodium and chloride ions. This compound also affects gene expression by altering the activity of transcription factors and other regulatory proteins . Furthermore, it impacts cellular metabolism by modulating the activity of enzymes involved in energy production and ion transport .
Molecular Mechanism
At the molecular level, this compound exerts its effects by binding to the chloride-binding site of the NKCC2 transporter in the thick ascending limb of the loop of Henle . This binding inhibits the cotransporter’s function, preventing the reabsorption of sodium, chloride, and potassium ions . Additionally, this compound interacts with organic anion transporters (OAT1) in the proximal tubule, facilitating its secretion into the tubular lumen . These interactions lead to increased excretion of these ions in the urine, resulting in diuresis and reduced fluid retention .
Temporal Effects in Laboratory Settings
In laboratory settings, the effects of this compound can change over time. The compound is relatively stable, but its efficacy may decrease with prolonged exposure due to the development of diuretic resistance . This resistance can result from adaptive changes in the expression and activity of NKCC2 and other transporters . Additionally, this compound can undergo degradation, leading to reduced potency and altered pharmacokinetics . Long-term studies have shown that chronic administration of this compound can lead to changes in renal function and electrolyte balance .
Dosage Effects in Animal Models
The effects of this compound vary with different dosages in animal models. At low doses, it effectively induces diuresis without significant adverse effects . At higher doses, this compound can cause electrolyte imbalances, dehydration, and renal dysfunction . In some cases, high doses can lead to ototoxicity and hearing loss . The threshold for these adverse effects varies between species, with cats being more susceptible than dogs .
Metabolic Pathways
This compound is primarily metabolized in the liver and kidneys through glucuronidation . It is extensively bound to plasma proteins, limiting its filtration by the glomerulus . Instead, it is secreted into the tubular lumen via organic anion transporters (OAT1) in the proximal tubule . This secretion allows this compound to reach its target sites in the loop of Henle, where it inhibits the NKCC2 transporter . The compound is then excreted in the urine, with a portion undergoing hepatic metabolism and biliary excretion .
Transport and Distribution
This compound is transported and distributed within cells and tissues through various mechanisms. It is highly bound to plasma proteins, primarily albumin, which limits its filtration by the glomerulus . Instead, it is actively secreted into the tubular lumen via organic anion transporters (OAT1) in the proximal tubule . This secretion allows this compound to reach its target sites in the loop of Henle, where it inhibits the NKCC2 transporter . The compound is then excreted in the urine, with a portion undergoing hepatic metabolism and biliary excretion .
Subcellular Localization
This compound’s subcellular localization is primarily within the renal tubular cells, particularly in the thick ascending limb of the loop of Henle . It binds to the NKCC2 transporter on the luminal membrane, inhibiting its function and preventing the reabsorption of sodium, chloride, and potassium ions . This localization is facilitated by the compound’s interaction with organic anion transporters (OAT1) in the proximal tubule, which secrete it into the tubular lumen . The subcellular localization of this compound is crucial for its diuretic effects and its ability to modulate renal function .
Preparation Methods
Synthetic Routes and Reaction Conditions
Furosemide sodium can be synthesized through a multi-step process. One common method involves the reaction of 4-chloro-5-sulfamoylanthranilic acid with furan-2-ylmethanamine in the presence of sodium methoxide and dimethyl sulfoxide at elevated temperatures (125-135°C) . This reaction yields furosemide, which can then be converted to its sodium salt form.
Industrial Production Methods
In industrial settings, furosemide sodium is often produced in large quantities using similar synthetic routes. The process typically involves the use of high-purity reagents and controlled reaction conditions to ensure the consistency and quality of the final product. The compound is then purified and formulated into various dosage forms, including tablets and injectable solutions .
Chemical Reactions Analysis
Types of Reactions
Furosemide sodium undergoes several types of chemical reactions, including:
Oxidation: Furosemide sodium can be oxidized under certain conditions, leading to the formation of various degradation products.
Reduction: The compound can also undergo reduction reactions, although these are less common.
Substitution: Furosemide sodium can participate in substitution reactions, particularly involving its functional groups such as the sulfonamide and carboxylic acid groups
Common Reagents and Conditions
Common reagents used in the reactions of furosemide sodium include oxidizing agents like hydrogen peroxide and reducing agents such as sodium borohydride. Reaction conditions vary depending on the desired outcome but often involve controlled temperatures and pH levels .
Major Products Formed
The major products formed from the reactions of furosemide sodium depend on the specific reaction conditions. For example, oxidation can lead to the formation of sulfonic acids, while substitution reactions can yield various derivatives with modified functional groups .
Scientific Research Applications
Furosemide sodium has a wide range of scientific research applications, including:
Chemistry: It is used as a model compound in studies of diuretic mechanisms and drug interactions.
Biology: Furosemide sodium is employed in research on renal function and electrolyte balance.
Medicine: The compound is extensively studied for its therapeutic effects in treating edema and hypertension.
Industry: Furosemide sodium is used in the development of new diuretic formulations and drug delivery systems .
Comparison with Similar Compounds
Similar Compounds
Furosemide sodium is often compared with other loop diuretics, such as:
- Bumetanide
- Torasemide
- Ethacrynic acid
Uniqueness
Compared to these similar compounds, furosemide sodium is unique in its rapid onset of action and relatively short duration of effect. It is particularly effective in acute settings where rapid diuresis is required. Additionally, furosemide sodium has a well-established safety profile and is widely used in clinical practice .
Properties
CAS No. |
41733-55-5 |
---|---|
Molecular Formula |
C12H11ClN2NaO5S |
Molecular Weight |
353.73 g/mol |
IUPAC Name |
sodium;4-chloro-2-(furan-2-ylmethylamino)-5-sulfamoylbenzoate |
InChI |
InChI=1S/C12H11ClN2O5S.Na/c13-9-5-10(15-6-7-2-1-3-20-7)8(12(16)17)4-11(9)21(14,18)19;/h1-5,15H,6H2,(H,16,17)(H2,14,18,19); |
InChI Key |
RSDYORUXBHNXHC-UHFFFAOYSA-N |
SMILES |
C1=COC(=C1)CNC2=CC(=C(C=C2C(=O)[O-])S(=O)(=O)N)Cl.[Na+] |
Canonical SMILES |
C1=COC(=C1)CNC2=CC(=C(C=C2C(=O)O)S(=O)(=O)N)Cl.[Na] |
41733-55-5 | |
Pictograms |
Health Hazard |
Related CAS |
54-31-9 (Parent) |
Origin of Product |
United States |
Retrosynthesis Analysis
AI-Powered Synthesis Planning: Our tool employs the Template_relevance Pistachio, Template_relevance Bkms_metabolic, Template_relevance Pistachio_ringbreaker, Template_relevance Reaxys, Template_relevance Reaxys_biocatalysis model, leveraging a vast database of chemical reactions to predict feasible synthetic routes.
One-Step Synthesis Focus: Specifically designed for one-step synthesis, it provides concise and direct routes for your target compounds, streamlining the synthesis process.
Accurate Predictions: Utilizing the extensive PISTACHIO, BKMS_METABOLIC, PISTACHIO_RINGBREAKER, REAXYS, REAXYS_BIOCATALYSIS database, our tool offers high-accuracy predictions, reflecting the latest in chemical research and data.
Strategy Settings
Precursor scoring | Relevance Heuristic |
---|---|
Min. plausibility | 0.01 |
Model | Template_relevance |
Template Set | Pistachio/Bkms_metabolic/Pistachio_ringbreaker/Reaxys/Reaxys_biocatalysis |
Top-N result to add to graph | 6 |
Feasible Synthetic Routes
Disclaimer and Information on In-Vitro Research Products
Please be aware that all articles and product information presented on BenchChem are intended solely for informational purposes. The products available for purchase on BenchChem are specifically designed for in-vitro studies, which are conducted outside of living organisms. In-vitro studies, derived from the Latin term "in glass," involve experiments performed in controlled laboratory settings using cells or tissues. It is important to note that these products are not categorized as medicines or drugs, and they have not received approval from the FDA for the prevention, treatment, or cure of any medical condition, ailment, or disease. We must emphasize that any form of bodily introduction of these products into humans or animals is strictly prohibited by law. It is essential to adhere to these guidelines to ensure compliance with legal and ethical standards in research and experimentation.