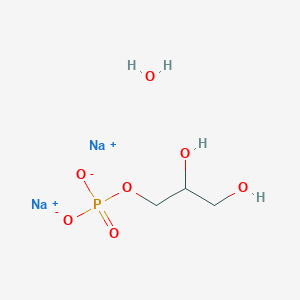
Glycerol phosphate disodium salt hydrate
Overview
Description
Mechanism of Action
Target of Action
The primary target of Sodium Glycerophosphate Hydrate is the serum alkaline phosphatases in the body . These enzymes play a crucial role in the hydrolysis of Sodium Glycerophosphate, which is essential for its function .
Mode of Action
Sodium Glycerophosphate acts as a donor of inorganic phosphate . It is hydrolyzed to inorganic phosphate and glycerol in the body . This reaction is dependent on the activity of serum alkaline phosphatases . The inorganic phosphate produced through this process plays a vital role in various biochemical processes in the body .
Biochemical Pathways
Sodium Glycerophosphate is involved in the phosphorylation process , one of the most common protein posttranslational modifications . It acts as a phosphate group donor in matrix mineralization studies and accelerates calcification in vascular smooth muscle cells . It also promotes bone matrix mineralization when delivered to osteoblasts, providing a crucial source of phosphate ions .
Pharmacokinetics
The pharmacokinetic properties of Sodium Glycerophosphate are characterized by its absorption and metabolism. After administration, the peak serum phosphate concentration is reached in about 4 hours . Glycerophosphate is hydrolyzed to form inorganic phosphate, and the extent of this reaction is dependent on serum alkaline phosphatase activity . The inorganic phosphate produced is eliminated in the urine . There may be a very small amount of glycerophosphate excreted in the urine unchanged .
Result of Action
The hydrolysis of Sodium Glycerophosphate results in the production of inorganic phosphate and glycerol . The inorganic phosphate produced plays a crucial role in various biochemical processes, including energy metabolism and signal transduction . It also contributes to the mineralization of bone matrix when delivered to osteoblasts .
Action Environment
The action of Sodium Glycerophosphate can be influenced by various environmental factors. For instance, the activity of serum alkaline phosphatases, which is crucial for the hydrolysis of Sodium Glycerophosphate, can be affected by factors such as pH and temperature . Furthermore, the solubility of Sodium Glycerophosphate in water suggests that its action, efficacy, and stability can be influenced by the hydration status of the body .
Biochemical Analysis
Biochemical Properties
Glycerol phosphate disodium salt hydrate plays a crucial role in biochemical reactions as a phosphate group donor. It is extensively used in kinase reaction buffers as a serine-threonine phosphatase inhibitor, providing broad-spectrum inhibition . This compound interacts with enzymes such as alkaline phosphatase, promoting bone matrix mineralization when delivered to osteoblasts . Additionally, it is used in culture media for mesenchymal stem cell differentiation to osteoblast-type cells .
Cellular Effects
This compound has significant effects on various cell types and cellular processes. It acts as a phosphate group donor in matrix mineralization studies, accelerating calcification in vascular smooth muscle cells . This compound also promotes bone matrix mineralization in osteoblasts, providing a crucial source of phosphate ions . Furthermore, it influences cell signaling pathways and gene expression, particularly in the differentiation of mesenchymal stem cells to osteoblasts .
Molecular Mechanism
At the molecular level, this compound functions as a potent protein phosphatase inhibitor, removing phosphate groups from phosphorylated amino acid residues of target proteins . This inhibition plays a vital role in regulating protein phosphorylation, one of the most common post-translational modifications. The compound also promotes bone matrix mineralization by providing a source of phosphate ions, which are essential for the calcification process .
Temporal Effects in Laboratory Settings
In laboratory settings, the effects of this compound can change over time. The compound is stable under standard storage conditions and maintains its activity in culture media . Long-term studies have shown that it continues to promote mineralization and differentiation of stem cells over extended periods . Its stability and degradation in different experimental conditions may vary, affecting its long-term impact on cellular functions.
Dosage Effects in Animal Models
The effects of this compound vary with different dosages in animal models. At optimal dosages, it promotes bone mineralization and calcification without adverse effects . At higher doses, it may lead to toxic effects and disrupt normal cellular functions . It is crucial to determine the appropriate dosage to achieve the desired outcomes while minimizing potential adverse effects.
Metabolic Pathways
This compound is involved in several metabolic pathways. It serves as a substrate for glycerol-3-phosphate dehydrogenase, which catalyzes the conversion of glycerol-3-phosphate to dihydroxyacetone phosphate (DHAP) . This reaction is a key step in glycolysis and gluconeogenesis, linking carbohydrate and lipid metabolism. The compound also interacts with enzymes such as glycerol kinase and triose phosphate isomerase, further integrating into metabolic pathways .
Transport and Distribution
Within cells and tissues, this compound is transported and distributed through specific transporters and binding proteins. It is readily soluble in water, facilitating its uptake and distribution in cellular environments . The compound’s localization and accumulation are influenced by its interactions with cellular transport mechanisms, ensuring its availability for biochemical reactions .
Subcellular Localization
This compound exhibits specific subcellular localization, which affects its activity and function. It is primarily localized in the cytoplasm, where it participates in metabolic pathways and biochemical reactions . The compound’s targeting signals and post-translational modifications direct it to specific compartments or organelles, ensuring its proper function within the cell .
Preparation Methods
Synthetic Routes and Reaction Conditions
Glycerol phosphate disodium salt hydrate can be synthesized through the reaction of glycerol with phosphoric acid, followed by neutralization with sodium hydroxide. The reaction typically involves the following steps:
Glycerol and Phosphoric Acid Reaction: Glycerol is reacted with phosphoric acid to form glycerol phosphate.
Neutralization: The resulting glycerol phosphate is then neutralized with sodium hydroxide to form glycerol phosphate disodium salt.
Hydration: The final product is obtained by crystallizing the compound in the presence of water to form the hydrate.
Industrial Production Methods
Industrial production of this compound involves similar steps but on a larger scale. The process includes:
Continuous Stirred Tank Reactors (CSTRs): The reaction is carried out in CSTRs to ensure uniform mixing and reaction conditions.
Filtration and Crystallization: The product is filtered to remove impurities and then crystallized to obtain the hydrate form.
Chemical Reactions Analysis
Types of Reactions
Glycerol phosphate disodium salt hydrate undergoes various chemical reactions, including:
Hydrolysis: It can be hydrolyzed to form glycerol and inorganic phosphate.
Oxidation: It can undergo oxidation reactions to form glyceraldehyde phosphate.
Substitution: It can participate in substitution reactions where the phosphate group is replaced by other functional groups.
Common Reagents and Conditions
Hydrolysis: Typically carried out in the presence of water and enzymes like alkaline phosphatase.
Oxidation: Requires oxidizing agents such as hydrogen peroxide or potassium permanganate.
Substitution: Involves reagents like alkyl halides or acyl chlorides under basic conditions.
Major Products
Hydrolysis: Glycerol and inorganic phosphate.
Oxidation: Glyceraldehyde phosphate.
Substitution: Various substituted glycerol derivatives depending on the reagents used.
Scientific Research Applications
Glycerol phosphate disodium salt hydrate has numerous applications in scientific research:
Cell Biology: It serves as a phosphate group donor in matrix mineralization studies and accelerates calcification in vascular smooth muscle cells.
Biochemical Research: It functions as a serine-threonine phosphatase inhibitor in kinase reaction buffers, offering broad-spectrum inhibition.
Metabolomics: It is used as an organic phosphate donor in culture media for mesenchymal stem cell differentiation to osteoblast-type cells.
Drug Delivery: It is utilized in chitosan hydrogel formulations for drug delivery research.
Recombinant Protein Production: It serves as a phosphate source for cell growth and recombinant protein production.
Micro-Arc Oxidation: It finds applications in micro-arc oxidation electrolyte solutions, contributing to the creation of photocatalytic coatings.
Comparison with Similar Compounds
Glycerol phosphate disodium salt hydrate can be compared with other similar compounds such as:
Glycerol 1-phosphate sodium salt hydrate: Similar in structure but differs in the position of the phosphate group.
DL-α-Glycerol phosphate magnesium salt hydrate: Contains magnesium instead of sodium.
sn-Glycerol 3-phosphate lithium salt: Contains lithium instead of sodium.
Uniqueness
This compound is unique due to its broad-spectrum inhibition of serine-threonine phosphatases and its extensive use as a phosphate donor in various biochemical and cell biology applications .
Properties
IUPAC Name |
disodium;2,3-dihydroxypropyl phosphate;hydrate | |
---|---|---|
Source | PubChem | |
URL | https://pubchem.ncbi.nlm.nih.gov | |
Description | Data deposited in or computed by PubChem | |
InChI |
InChI=1S/C3H9O6P.2Na.H2O/c4-1-3(5)2-9-10(6,7)8;;;/h3-5H,1-2H2,(H2,6,7,8);;;1H2/q;2*+1;/p-2 | |
Source | PubChem | |
URL | https://pubchem.ncbi.nlm.nih.gov | |
Description | Data deposited in or computed by PubChem | |
InChI Key |
OFNNKPAERNWEDD-UHFFFAOYSA-L | |
Source | PubChem | |
URL | https://pubchem.ncbi.nlm.nih.gov | |
Description | Data deposited in or computed by PubChem | |
Canonical SMILES |
C(C(COP(=O)([O-])[O-])O)O.O.[Na+].[Na+] | |
Source | PubChem | |
URL | https://pubchem.ncbi.nlm.nih.gov | |
Description | Data deposited in or computed by PubChem | |
Molecular Formula |
C3H9Na2O7P | |
Source | PubChem | |
URL | https://pubchem.ncbi.nlm.nih.gov | |
Description | Data deposited in or computed by PubChem | |
Molecular Weight |
234.05 g/mol | |
Source | PubChem | |
URL | https://pubchem.ncbi.nlm.nih.gov | |
Description | Data deposited in or computed by PubChem | |
CAS No. |
55073-41-1 | |
Record name | Sodium glycerophosphate [USAN:NF] | |
Source | ChemIDplus | |
URL | https://pubchem.ncbi.nlm.nih.gov/substance/?source=chemidplus&sourceid=0055073411 | |
Description | ChemIDplus is a free, web search system that provides access to the structure and nomenclature authority files used for the identification of chemical substances cited in National Library of Medicine (NLM) databases, including the TOXNET system. | |
Retrosynthesis Analysis
AI-Powered Synthesis Planning: Our tool employs the Template_relevance Pistachio, Template_relevance Bkms_metabolic, Template_relevance Pistachio_ringbreaker, Template_relevance Reaxys, Template_relevance Reaxys_biocatalysis model, leveraging a vast database of chemical reactions to predict feasible synthetic routes.
One-Step Synthesis Focus: Specifically designed for one-step synthesis, it provides concise and direct routes for your target compounds, streamlining the synthesis process.
Accurate Predictions: Utilizing the extensive PISTACHIO, BKMS_METABOLIC, PISTACHIO_RINGBREAKER, REAXYS, REAXYS_BIOCATALYSIS database, our tool offers high-accuracy predictions, reflecting the latest in chemical research and data.
Strategy Settings
Precursor scoring | Relevance Heuristic |
---|---|
Min. plausibility | 0.01 |
Model | Template_relevance |
Template Set | Pistachio/Bkms_metabolic/Pistachio_ringbreaker/Reaxys/Reaxys_biocatalysis |
Top-N result to add to graph | 6 |
Feasible Synthetic Routes
Q1: What makes Glycerol Phosphate Disodium Salt Hydrate suitable for creating in situ gelling systems for drug delivery?
A1: this compound exhibits excellent biocompatibility and can be combined with polymers like chitosan to create in situ forming gels. [, ] This means that the mixture remains in a liquid state at room temperature, but upon injection into the body (reaching physiological temperature and pH), it transforms into a gel. This property is incredibly valuable for delivering drugs subcutaneously, offering controlled release and potentially improved patient comfort compared to traditional injections. []
Q2: How does the molecular weight of chitosan influence its interaction with this compound in gel formation?
A2: Research indicates that the molecular weight of chitosan plays a crucial role in determining the sol-gel transition temperature of the mixture. [] Chitosan with varying molecular weights, when combined with this compound, resulted in gels that transitioned from liquid to gel state within the range of 31°C to 37°C. This suggests that by carefully selecting the appropriate molecular weight of chitosan, one can fine-tune the gelling temperature, enabling customized drug release profiles. []
Q3: What analytical techniques are commonly employed to characterize the properties of this compound-based formulations?
A3: Various analytical methods are utilized to ensure the quality and effectiveness of these formulations. Researchers often measure properties like pH, drug content, sedimentation volume, and viscosity. [] Techniques like particle size analysis and zeta potential measurements help determine the stability and drug release characteristics of the formulation. In vitro drug release studies are also crucial for understanding the release kinetics of the incorporated drug from the gel matrix. []
Disclaimer and Information on In-Vitro Research Products
Please be aware that all articles and product information presented on BenchChem are intended solely for informational purposes. The products available for purchase on BenchChem are specifically designed for in-vitro studies, which are conducted outside of living organisms. In-vitro studies, derived from the Latin term "in glass," involve experiments performed in controlled laboratory settings using cells or tissues. It is important to note that these products are not categorized as medicines or drugs, and they have not received approval from the FDA for the prevention, treatment, or cure of any medical condition, ailment, or disease. We must emphasize that any form of bodily introduction of these products into humans or animals is strictly prohibited by law. It is essential to adhere to these guidelines to ensure compliance with legal and ethical standards in research and experimentation.