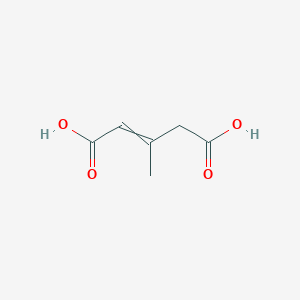
3-Methylglutaconic acid
Overview
Description
3-Methylglutaconic acid (3-MGA) is a branched-chain organic acid (C₆H₈O₄) involved in mitochondrial energy metabolism, particularly in leucine catabolism. It exists as a conjugate acid with two carboxylic acid groups and a methyl-substituted double bond. 3-MGA is primarily associated with inborn errors of metabolism (IEMs) characterized by mitochondrial dysfunction . Elevated urinary excretion of 3-MGA, termed 3-methylglutaconic aciduria, is a hallmark of disorders such as:
- Barth syndrome (BTHS)
- 3-Methylglutaconyl-CoA hydratase deficiency (primary 3-MGA-uria, Type I)
- Costeff syndrome (Type III)
- Mitochondrial encephalopathies (Type IV)
The acid’s accumulation arises from either:
Primary defects in leucine degradation (e.g., 3-methylglutaconyl-CoA hydratase deficiency) , or
Secondary mitochondrial dysfunction (e.g., impaired Krebs cycle or oxidative phosphorylation) .
Preparation Methods
Synthetic Routes and Reaction Conditions: The synthesis of 3-methylglutaconic acid typically involves the reaction of 3-methylglutaconyl-CoA with water, catalyzed by the enzyme 3-methylglutaconyl-CoA hydratase . This reaction occurs under physiological conditions within the mitochondria.
Industrial Production Methods: Industrial production methods for this compound are not well-documented, likely due to its primary occurrence as a metabolic intermediate rather than a commercially produced compound. it can be synthesized in the laboratory using standard organic synthesis techniques involving the appropriate precursors and catalysts.
Chemical Reactions Analysis
Enzyme-Catalyzed Reactions
Enzyme | Reaction Catalyzed | EC Number |
---|---|---|
3-Methylglutaconyl-CoA hydratase | Reversible hydration of HMG-CoA ↔ 3-methylglutaconyl-CoA | 4.2.1.18 |
Acetoacetyl-CoA thiolase | Condensation of 2 acetyl-CoA → acetoacetyl-CoA | 2.3.1.9 |
HMG-CoA synthase 2 | Acetoacetyl-CoA + acetyl-CoA → HMG-CoA | 2.3.3.10 |
3-Methylcrotonyl-CoA carboxylase | 3-Methylcrotonyl-CoA → 3-methylglutaconyl-CoA | 6.4.1.4 |
Non-Enzymatic Isomerization
3-Methylglutaconyl-CoA undergoes spontaneous intramolecular cyclization, producing cis-3MGA (major) and trans-3MGA (minor) :
-
Cyclization : HMG-CoA → HMG anhydride + CoASH (non-enzymatic)
-
Hydration : HMG anhydride ↔ cis/trans-3MGA
Isomer Detection
Diastereomer | Detection Method | Source |
---|---|---|
cis-3MGA | Competitive immunoassay using α-3MGC IgG | Urine of patients with 3MGA-uria |
trans-3MGA | GC-MS analysis | In vitro assays with AUH enzyme |
Key Research Findings
-
Mitochondrial Dysfunction Link : Secondary 3MGA accumulation occurs due to electron transport chain defects, diverting acetyl-CoA into the mevalonate shunt .
-
Hydratase Reversibility : 3-Methylglutaconyl-CoA hydratase (AUH) catalyzes HMG-CoA dehydration to 3-methylglutaconyl-CoA under metabolic stress .
-
Thioesterase Specificity : Acyl-CoA thioesterases (ACOT4/8) hydrolyze 3-methylglutaconyl-CoA, with tissue-specific expression influencing 3-MGA levels .
-
Diastereomer Specificity : cis-3MGA predominates in human urine due to non-enzymatic isomerization of trans-3MGA-CoA .
Pathological Implications
-
Acidogen Effects : Elevated 3-MGA disrupts cellular pH, causing metabolic acidosis .
-
Metabotoxin Activity : Chronic accumulation inhibits mitochondrial enzymes, exacerbating energy deficits .
This synthesis integrates enzymatic pathways, isomerization dynamics, and clinical biochemistry to elucidate 3-MGA's chemical reactivity. Experimental data from hydratase assays, isotopic tracing, and immunoassays validate these mechanisms .
Scientific Research Applications
3-Methylglutaconic acid has several scientific research applications, including:
Chemistry: It is studied as an intermediate in metabolic pathways and for its role in various biochemical reactions.
Biology: Research focuses on its accumulation in metabolic disorders and its role in mitochondrial function.
Industry: While not widely used industrially, its derivatives and related compounds may have applications in biochemical research and diagnostics.
Mechanism of Action
The mechanism of action of 3-methylglutaconic acid involves its role as an intermediate in the leucine catabolism pathway and the mevalonate shunt . It is converted to other metabolites through enzymatic reactions, primarily involving 3-methylglutaconyl-CoA hydratase. This enzyme catalyzes the hydration of 3-methylglutaconyl-CoA to form 3-hydroxy-3-methylglutaryl-CoA, which is further metabolized in the mitochondria .
Comparison with Similar Compounds
Comparison with Structurally and Functionally Related Compounds
3-MGA is often measured alongside similar organic acids in clinical diagnostics. Below is a detailed comparison:
3-Methylglutaric Acid (3-MG)
Key Distinction: 3-MG is a stable byproduct of 3-MGA decarboxylation, often co-detected in urine. However, 3-MG lacks diagnostic specificity compared to 3-MGA .
Glutaric Acid (GA)
Key Distinction : GA lacks the methyl group and double bond of 3-MGA, leading to distinct metabolic implications.
2-Hydroxyisovaleric Acid (2-HIVA)
3-Hydroxyglutaric Acid (3-HGA)
Key Distinction : 3-HGA’s hydroxyl group differentiates its metabolic pathway and clinical implications from 3-MGA.
Biochemical and Diagnostic Insights
Isomer-Specific Detection
3-MGA exists in cis and trans isoforms, detectable via NMR or GC-MS. In Type I 3-MGA-uria, the cis:trans ratio is 2:1, while Type IV shows a 1:1 ratio .
Metabolomic Profiling
- Primary 3-MGA-uria (Type I) : Elevated 3-MGA, 3-MG, and 3-hydroxyisovaleric acid (3-HIVA) due to 3-MGH deficiency .
- Secondary 3-MGA-uria (Types II–IV): Isolated 3-MGA elevation with normal 3-HIVA levels .
Genetic and Enzymatic Differentiation
Clinical and Research Implications
- Diagnostic Challenges: 3-MGA’s structural similarity to other acids necessitates advanced metabolomics (GC-MS, 2D-NMR) .
- Therapeutic Targets : Bezafibrate reduces 3-MGA toxicity in mitochondrial models , while dietary leucine restriction is ineffective in secondary forms .
Biological Activity
3-Methylglutaconic acid (MGA) is a significant organic acid that plays a role in various metabolic pathways, particularly in the degradation of leucine. Its biological activity is linked to several metabolic disorders, primarily those affecting mitochondrial function. This article presents a comprehensive overview of the biological activity of this compound, including its biochemical implications, associated syndromes, and relevant case studies.
Biochemical Pathways
This compound is an intermediate in the leucine catabolism pathway. It is produced through the action of 3-methylglutaconyl CoA hydratase, which converts HMG CoA into 3-methylglutaconyl CoA. This reaction is crucial for the proper metabolism of leucine, an essential amino acid. The accumulation of MGA can indicate disruptions in these metabolic pathways, particularly under conditions where mitochondrial function is compromised.
Table 1: Key Enzymatic Reactions Involving this compound
Enzyme | Reaction Description | Role in Metabolism |
---|---|---|
3-Methylglutaconyl CoA hydratase | Converts HMG CoA to 3-methylglutaconyl CoA | Key step in leucine degradation |
Acetoacetyl CoA thiolase | Condenses two acetyl CoA into acetoacetyl CoA | Initiates pathway leading to MGA formation |
3-Methylcrotonyl CoA carboxylase | Utilizes ATP to convert 3-methylcrotonyl CoA to HMG CoA | Important for leucine catabolism |
Biological Activity and Mechanisms
Research indicates that elevated levels of this compound are associated with various mitochondrial disorders. The biological activity of MGA includes:
- Inhibition of Mitochondrial Function : Studies have shown that MGA can compromise mitochondrial bioenergetics by reducing mitochondrial redox potential and inhibiting critical enzymes such as Na,K-ATPase. This inhibition may lead to increased oxidative stress within cells, contributing to neurodegenerative processes .
- Reactive Species Production : The presence of MGA has been linked to increased production of reactive oxygen species (ROS), which can further damage cellular components and exacerbate mitochondrial dysfunction .
- Neurological Implications : Patients with elevated MGA levels often present with neurological symptoms, including spastic paraparesis and cognitive deficits, highlighting its potential role in neurodevelopmental disorders .
Case Studies and Clinical Findings
Several case studies have documented the clinical significance of 3-methylglutaconic aciduria (MGAuria), which is characterized by the urinary excretion of elevated levels of MGA.
-
Case Study: Mitochondrial Dysfunction
A study involving patients with mitochondrial disorders revealed that those with high levels of MGA exhibited symptoms such as Leigh syndrome and other neurodegenerative conditions. The biochemical analysis indicated a correlation between elevated MGA levels and respiratory chain deficiencies . -
Case Study: Genetic Mutations
In a cohort study focusing on patients with 3-methylglutaconic aciduria type IV, researchers identified specific genetic mutations that led to dysfunctional oxidative phosphorylation. The presence of MGA served as a biochemical marker for diagnosing these conditions .
Q & A
Basic Research Questions
Q. What clinical and metabolic implications are associated with elevated 3-methylglutaconic acid (3-MGA) levels?
Elevated 3-MGA is a biomarker for mitochondrial dysfunction and genetic disorders such as Barth syndrome, 3-methylglutaconic aciduria (MGA), and Leigh syndrome. Its accumulation correlates with impaired leucine metabolism and mitochondrial energy production, often presenting with symptoms like cardiomyopathy, skeletal myopathy, and developmental delays . Methodologically, researchers should integrate urine organic acid analysis (e.g., gas chromatography-mass spectrometry) with plasma amino acid profiling to confirm metabolic disturbances and rule out secondary causes like lactic acidosis .
Q. Which genetic disorders are most strongly linked to 3-MGAuria, and how are they diagnosed?
Primary disorders include Barth syndrome (TAZ gene mutations), MGA types I-VII (e.g., MGCA1, MGA4 mutations), and mitochondrial encephalopathies. Diagnosis requires urinary 3-MGA quantification, genetic testing (e.g., whole-exome sequencing), and functional assays of mitochondrial respiratory chain enzymes. Note that 3-MGA levels can vary intermittently, necessitating repeated sampling and correlation with clinical phenotypes .
Q. What analytical techniques are recommended for detecting 3-MGA in biological samples?
Liquid chromatography-tandem mass spectrometry (LC-MS/MS) is the gold standard due to its sensitivity in distinguishing 3-MGA from structurally similar metabolites like 3-methylglutaric acid. Researchers must include internal standards (e.g., deuterated 3-MGA) and validate assays against control populations to minimize false positives .
Q. How does 3-MGA correlate with mitochondrial dysfunction in experimental models?
In vitro studies using fibroblast cultures from patients with Barth syndrome show reduced cardiolipin remodeling and abnormal mitochondrial morphology. Researchers can measure respiratory chain complex activity (e.g., Complex IV) and ATP production rates to quantify dysfunction. Concurrently, 3-MGA levels in culture media provide a metabolic readout .
Advanced Research Questions
Q. What challenges arise in quantifying 3-MGA in heterogeneous patient populations, and how can they be mitigated?
Variability in urinary excretion (e.g., intermittent elevation in Barth syndrome) and overlapping metabolite profiles (e.g., 3-hydroxy-3-methylglutaric acid) complicate quantification. Researchers should employ longitudinal sampling, use age-matched controls, and apply multivariate statistical models (e.g., PCA) to distinguish pathological from physiological variations .
Q. How do genetic mutations in TAZ or MGCA1 alter 3-MGA metabolism, and what experimental models best capture these effects?
TAZ mutations disrupt cardiolipin synthesis, leading to mitochondrial membrane instability. Knockout murine models and induced pluripotent stem cell (iPSC)-derived cardiomyocytes are effective for studying tissue-specific metabolic cascades. Stable isotope tracing (e.g., ¹³C-leucine) can track 3-MGA flux in these systems .
Q. What subclassifications of 3-MGAuria exist based on metabolite profiles, and how do they inform therapeutic strategies?
Type IV MGA (severe psychomotor delay, neonatal hypotension) shows distinct 3-MGA/3-methylglutaric acid ratios compared to Type VII (lactic acidosis, hyperammonemia). Researchers should stratify patients using urinary metabolite panels and prioritize gene therapy or substrate reduction therapy based on enzymatic deficiencies (e.g., enoyl-CoA hydratase) .
Q. What experimental design considerations are critical for resolving contradictory data in 3-MGA studies?
Contradictions often stem from small sample sizes, inconsistent phenotyping, or methodological variability. Robust studies should:
- Use standardized protocols for sample collection (e.g., fasting vs. postprandial).
- Incorporate multi-omics approaches (metabolomics, proteomics) to identify confounding factors.
- Apply Bayesian statistics to assess the probability of hypotheses in underpowered cohorts .
Q. How can researchers differentiate primary 3-MGAuria from secondary elevations caused by other metabolic disturbances?
Secondary elevations (e.g., due to renal dysfunction or drug toxicity) lack genetic markers and show transient 3-MGAuria. Combine whole-genome sequencing with functional assays (e.g., mitochondrial membrane potential measurements) to confirm primary etiology. Cross-validate findings with databases like ClinVar or OMIM .
Properties
CAS No. |
5746-90-7 |
---|---|
Molecular Formula |
C6H8O4 |
Molecular Weight |
144.12 g/mol |
IUPAC Name |
3-methylpent-2-enedioic acid |
InChI |
InChI=1S/C6H8O4/c1-4(2-5(7)8)3-6(9)10/h2H,3H2,1H3,(H,7,8)(H,9,10) |
InChI Key |
WKRBKYFIJPGYQC-UHFFFAOYSA-N |
SMILES |
CC(=CC(=O)O)CC(=O)O |
Isomeric SMILES |
C/C(=C\C(=O)O)/CC(=O)O |
Canonical SMILES |
CC(=CC(=O)O)CC(=O)O |
Key on ui other cas no. |
5746-90-7 |
physical_description |
Solid |
Pictograms |
Irritant |
Synonyms |
3-Methyl-2-pentenedioic Acid; 3-Methyl-glutaconic Acid; NSC 249232; β-Methylglutaconic Acid |
Origin of Product |
United States |
Retrosynthesis Analysis
AI-Powered Synthesis Planning: Our tool employs the Template_relevance Pistachio, Template_relevance Bkms_metabolic, Template_relevance Pistachio_ringbreaker, Template_relevance Reaxys, Template_relevance Reaxys_biocatalysis model, leveraging a vast database of chemical reactions to predict feasible synthetic routes.
One-Step Synthesis Focus: Specifically designed for one-step synthesis, it provides concise and direct routes for your target compounds, streamlining the synthesis process.
Accurate Predictions: Utilizing the extensive PISTACHIO, BKMS_METABOLIC, PISTACHIO_RINGBREAKER, REAXYS, REAXYS_BIOCATALYSIS database, our tool offers high-accuracy predictions, reflecting the latest in chemical research and data.
Strategy Settings
Precursor scoring | Relevance Heuristic |
---|---|
Min. plausibility | 0.01 |
Model | Template_relevance |
Template Set | Pistachio/Bkms_metabolic/Pistachio_ringbreaker/Reaxys/Reaxys_biocatalysis |
Top-N result to add to graph | 6 |
Feasible Synthetic Routes
Disclaimer and Information on In-Vitro Research Products
Please be aware that all articles and product information presented on BenchChem are intended solely for informational purposes. The products available for purchase on BenchChem are specifically designed for in-vitro studies, which are conducted outside of living organisms. In-vitro studies, derived from the Latin term "in glass," involve experiments performed in controlled laboratory settings using cells or tissues. It is important to note that these products are not categorized as medicines or drugs, and they have not received approval from the FDA for the prevention, treatment, or cure of any medical condition, ailment, or disease. We must emphasize that any form of bodily introduction of these products into humans or animals is strictly prohibited by law. It is essential to adhere to these guidelines to ensure compliance with legal and ethical standards in research and experimentation.