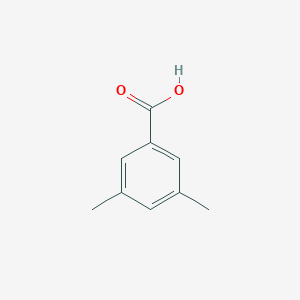
3,5-Dimethylbenzoic acid
Overview
Description
3,5-Dimethylbenzoic acid, also known as mesitylenic acid, is an organic compound with the molecular formula C₉H₁₀O₂. It is a derivative of benzoic acid, where two methyl groups are substituted at the 3rd and 5th positions of the benzene ring. This compound is known for its crystalline structure and is used in various chemical syntheses and industrial applications .
Mechanism of Action
Target of Action
3,5-Dimethylbenzoic acid is a synthetic compound that has been used in the synthesis of other organic compounds .
Mode of Action
It has been found to mimic the natural insect moulting hormones or ecdysteroids . These synthetic compounds introduce a new class of insect growth regulators (IGRs) exhibiting a novel mode of action, based on more insect-specific biochemical sites of action .
Biochemical Pathways
It is known that the compound mimics the ecdysteroid mode of action by true competitive binding on the ecdysteroid receptors (ecrs) . This suggests that it may affect the pathways involved in insect growth and development.
Result of Action
It has been shown to inhibit the growth of bacteria in vitro , suggesting that it may have antimicrobial properties.
Action Environment
It is recommended to keep the compound in a dry, cool, and well-ventilated place to maintain its stability.
Biochemical Analysis
Biochemical Properties
3,5-Dimethylbenzoic acid is known to form an inclusion complex with β-cyclodextrin . It is used as a carbon and energy supplement in the culture medium of Pseudomonas sp. WR912
Molecular Mechanism
It is known that the compound can form complexes with other molecules, such as β-cyclodextrin , but the specifics of these interactions and their effects at the molecular level are not clear.
Preparation Methods
Synthetic Routes and Reaction Conditions: 3,5-Dimethylbenzoic acid can be synthesized through the oxidation of mesitylene. The process involves mixing mesitylene with an acetic acid solvent and a cobalt acetate catalyst. Compressed air is then introduced into the oxidation container, leading to the formation of this compound. The reaction mixture is subsequently crystallized, filtered, and purified .
Industrial Production Methods: In industrial settings, the production of this compound follows a similar oxidation process. The reaction is carried out in large-scale reactors where mesitylene is oxidized using acetic acid and cobalt acetate as a catalyst. The reaction heat is managed by continuous evaporation and condensation, ensuring a stable temperature and high yield .
Chemical Reactions Analysis
Types of Reactions: 3,5-Dimethylbenzoic acid undergoes various chemical reactions, including:
Oxidation: It can be further oxidized to form 3,5-dimethylbenzaldehyde.
Reduction: The carboxylic acid group can be reduced to form the corresponding alcohol.
Substitution: The methyl groups can undergo electrophilic substitution reactions.
Common Reagents and Conditions:
Oxidation: Uses oxidizing agents like potassium permanganate or chromium trioxide.
Reduction: Utilizes reducing agents such as lithium aluminum hydride.
Substitution: Involves reagents like halogens or nitrating agents under acidic conditions.
Major Products Formed:
Oxidation: 3,5-Dimethylbenzaldehyde.
Reduction: 3,5-Dimethylbenzyl alcohol.
Substitution: Various substituted derivatives depending on the reagent used.
Scientific Research Applications
3,5-Dimethylbenzoic acid is used in several scientific research fields:
Chemistry: As a reagent in organic synthesis and as a precursor for various chemical compounds.
Biology: Used as a carbon and energy supplement in the culture medium of certain bacteria like Pseudomonas sp.
Medicine: Investigated for its potential use in drug synthesis and as an intermediate in pharmaceutical production.
Industry: Employed in the manufacture of pesticides, fire retardants, and softening agents.
Comparison with Similar Compounds
- 3,5-Dimethoxybenzoic acid
- 2,6-Dimethylbenzoyl chloride
- 3,5-Dimethylbenzoyl chloride
- 2,4,6-Trimethylbenzoic acid
- 3,5-Dichlorobenzoic acid
- 2,3-Dimethylbenzoic acid
Comparison: 3,5-Dimethylbenzoic acid is unique due to its specific substitution pattern, which influences its reactivity and applications. Compared to its analogs, it has distinct properties that make it suitable for specific industrial and research applications. For example, its methyl groups at the 3rd and 5th positions provide steric hindrance, affecting its reactivity in substitution reactions .
Properties
IUPAC Name |
3,5-dimethylbenzoic acid | |
---|---|---|
Source | PubChem | |
URL | https://pubchem.ncbi.nlm.nih.gov | |
Description | Data deposited in or computed by PubChem | |
InChI |
InChI=1S/C9H10O2/c1-6-3-7(2)5-8(4-6)9(10)11/h3-5H,1-2H3,(H,10,11) | |
Source | PubChem | |
URL | https://pubchem.ncbi.nlm.nih.gov | |
Description | Data deposited in or computed by PubChem | |
InChI Key |
UMVOQQDNEYOJOK-UHFFFAOYSA-N | |
Source | PubChem | |
URL | https://pubchem.ncbi.nlm.nih.gov | |
Description | Data deposited in or computed by PubChem | |
Canonical SMILES |
CC1=CC(=CC(=C1)C(=O)O)C | |
Source | PubChem | |
URL | https://pubchem.ncbi.nlm.nih.gov | |
Description | Data deposited in or computed by PubChem | |
Molecular Formula |
C9H10O2 | |
Source | PubChem | |
URL | https://pubchem.ncbi.nlm.nih.gov | |
Description | Data deposited in or computed by PubChem | |
DSSTOX Substance ID |
DTXSID4060101 | |
Record name | Benzoic acid, 3,5-dimethyl- | |
Source | EPA DSSTox | |
URL | https://comptox.epa.gov/dashboard/DTXSID4060101 | |
Description | DSSTox provides a high quality public chemistry resource for supporting improved predictive toxicology. | |
Molecular Weight |
150.17 g/mol | |
Source | PubChem | |
URL | https://pubchem.ncbi.nlm.nih.gov | |
Description | Data deposited in or computed by PubChem | |
CAS No. |
499-06-9 | |
Record name | 3,5-Dimethylbenzoic acid | |
Source | CAS Common Chemistry | |
URL | https://commonchemistry.cas.org/detail?cas_rn=499-06-9 | |
Description | CAS Common Chemistry is an open community resource for accessing chemical information. Nearly 500,000 chemical substances from CAS REGISTRY cover areas of community interest, including common and frequently regulated chemicals, and those relevant to high school and undergraduate chemistry classes. This chemical information, curated by our expert scientists, is provided in alignment with our mission as a division of the American Chemical Society. | |
Explanation | The data from CAS Common Chemistry is provided under a CC-BY-NC 4.0 license, unless otherwise stated. | |
Record name | 3,5-Dimethylbenzoic acid | |
Source | ChemIDplus | |
URL | https://pubchem.ncbi.nlm.nih.gov/substance/?source=chemidplus&sourceid=0000499069 | |
Description | ChemIDplus is a free, web search system that provides access to the structure and nomenclature authority files used for the identification of chemical substances cited in National Library of Medicine (NLM) databases, including the TOXNET system. | |
Record name | 3,5-DIMETHYLBENZOIC ACID | |
Source | DTP/NCI | |
URL | https://dtp.cancer.gov/dtpstandard/servlet/dwindex?searchtype=NSC&outputformat=html&searchlist=37562 | |
Description | The NCI Development Therapeutics Program (DTP) provides services and resources to the academic and private-sector research communities worldwide to facilitate the discovery and development of new cancer therapeutic agents. | |
Explanation | Unless otherwise indicated, all text within NCI products is free of copyright and may be reused without our permission. Credit the National Cancer Institute as the source. | |
Record name | Benzoic acid, 3,5-dimethyl- | |
Source | EPA Chemicals under the TSCA | |
URL | https://www.epa.gov/chemicals-under-tsca | |
Description | EPA Chemicals under the Toxic Substances Control Act (TSCA) collection contains information on chemicals and their regulations under TSCA, including non-confidential content from the TSCA Chemical Substance Inventory and Chemical Data Reporting. | |
Record name | Benzoic acid, 3,5-dimethyl- | |
Source | EPA DSSTox | |
URL | https://comptox.epa.gov/dashboard/DTXSID4060101 | |
Description | DSSTox provides a high quality public chemistry resource for supporting improved predictive toxicology. | |
Record name | 3,5-dimethylbenzoic acid | |
Source | European Chemicals Agency (ECHA) | |
URL | https://echa.europa.eu/substance-information/-/substanceinfo/100.007.161 | |
Description | The European Chemicals Agency (ECHA) is an agency of the European Union which is the driving force among regulatory authorities in implementing the EU's groundbreaking chemicals legislation for the benefit of human health and the environment as well as for innovation and competitiveness. | |
Explanation | Use of the information, documents and data from the ECHA website is subject to the terms and conditions of this Legal Notice, and subject to other binding limitations provided for under applicable law, the information, documents and data made available on the ECHA website may be reproduced, distributed and/or used, totally or in part, for non-commercial purposes provided that ECHA is acknowledged as the source: "Source: European Chemicals Agency, http://echa.europa.eu/". Such acknowledgement must be included in each copy of the material. ECHA permits and encourages organisations and individuals to create links to the ECHA website under the following cumulative conditions: Links can only be made to webpages that provide a link to the Legal Notice page. | |
Record name | 3,5-DIMETHYLBENZOIC ACID | |
Source | FDA Global Substance Registration System (GSRS) | |
URL | https://gsrs.ncats.nih.gov/ginas/app/beta/substances/ED8AV34N0Y | |
Description | The FDA Global Substance Registration System (GSRS) enables the efficient and accurate exchange of information on what substances are in regulated products. Instead of relying on names, which vary across regulatory domains, countries, and regions, the GSRS knowledge base makes it possible for substances to be defined by standardized, scientific descriptions. | |
Explanation | Unless otherwise noted, the contents of the FDA website (www.fda.gov), both text and graphics, are not copyrighted. They are in the public domain and may be republished, reprinted and otherwise used freely by anyone without the need to obtain permission from FDA. Credit to the U.S. Food and Drug Administration as the source is appreciated but not required. | |
Synthesis routes and methods
Procedure details
Retrosynthesis Analysis
AI-Powered Synthesis Planning: Our tool employs the Template_relevance Pistachio, Template_relevance Bkms_metabolic, Template_relevance Pistachio_ringbreaker, Template_relevance Reaxys, Template_relevance Reaxys_biocatalysis model, leveraging a vast database of chemical reactions to predict feasible synthetic routes.
One-Step Synthesis Focus: Specifically designed for one-step synthesis, it provides concise and direct routes for your target compounds, streamlining the synthesis process.
Accurate Predictions: Utilizing the extensive PISTACHIO, BKMS_METABOLIC, PISTACHIO_RINGBREAKER, REAXYS, REAXYS_BIOCATALYSIS database, our tool offers high-accuracy predictions, reflecting the latest in chemical research and data.
Strategy Settings
Precursor scoring | Relevance Heuristic |
---|---|
Min. plausibility | 0.01 |
Model | Template_relevance |
Template Set | Pistachio/Bkms_metabolic/Pistachio_ringbreaker/Reaxys/Reaxys_biocatalysis |
Top-N result to add to graph | 6 |
Feasible Synthetic Routes
Disclaimer and Information on In-Vitro Research Products
Please be aware that all articles and product information presented on BenchChem are intended solely for informational purposes. The products available for purchase on BenchChem are specifically designed for in-vitro studies, which are conducted outside of living organisms. In-vitro studies, derived from the Latin term "in glass," involve experiments performed in controlled laboratory settings using cells or tissues. It is important to note that these products are not categorized as medicines or drugs, and they have not received approval from the FDA for the prevention, treatment, or cure of any medical condition, ailment, or disease. We must emphasize that any form of bodily introduction of these products into humans or animals is strictly prohibited by law. It is essential to adhere to these guidelines to ensure compliance with legal and ethical standards in research and experimentation.