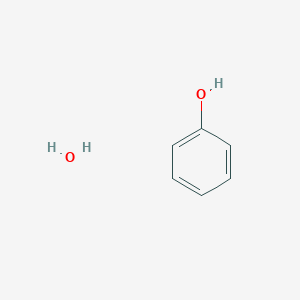
Phenol hydrate
- Click on QUICK INQUIRY to receive a quote from our team of experts.
- With the quality product at a COMPETITIVE price, you can focus more on your research.
Overview
Description
Phenol hydrate is a useful research compound. Its molecular formula is C6H8O2 and its molecular weight is 112.13 g/mol. The purity is usually 95%.
BenchChem offers high-quality this compound suitable for many research applications. Different packaging options are available to accommodate customers' requirements. Please inquire for more information about this compound including the price, delivery time, and more detailed information at info@benchchem.com.
Scientific Research Applications
Chemical Synthesis and Industrial Applications
Phenol hydrate serves as a crucial intermediate in the synthesis of various chemical compounds. Its primary applications include:
- Production of Bisphenol A : this compound is used in the synthesis of bisphenol A, which is essential for producing polycarbonates and epoxy resins. This application represents a significant portion of phenol's industrial use .
- Phenolic Resins : The reaction of phenol with formaldehyde leads to the formation of phenolic resins, widely used in adhesives, coatings, and plastics. These resins are known for their durability and heat resistance .
- Pharmaceuticals : In medicinal chemistry, this compound is utilized in the synthesis of various pharmaceuticals, including antiseptics and analgesics. Its hydroxyl group contributes to the biological activity of these compounds .
Environmental Applications
This compound plays a role in environmental science, particularly in the treatment of wastewater containing phenolic compounds:
- Wastewater Treatment : A case study demonstrated the effective removal of phenol from wastewater using advanced oxidation processes. In one instance, phenol concentrations were reduced from 4,761 mg/L to 1,096 mg/L through chemical treatment methods . This showcases the potential for this compound in mitigating environmental pollution.
Biological Interactions
This compound exhibits unique interactions with biological systems:
- DNA Extraction : In molecular biology laboratories, this compound is often used in liquid-liquid extraction protocols to isolate nucleic acids from biological samples. The compound facilitates the separation of nucleic acids from proteins and other contaminants .
- Toxicological Studies : Research has indicated that phenol and its hydrates can exhibit developmental and reproductive toxicity at high concentrations. Studies involving animal models have shown adverse effects related to exposure to phenolic compounds, highlighting the need for careful management in industrial applications .
Photochemical Applications
Recent studies have explored the photochemical properties of this compound:
- Photochemical Reactions : Phenol exhibits unique photochemical behavior at the air-water interface, where its hydration state influences reaction kinetics significantly. Research indicates that the photoionization rates of phenol are markedly enhanced when hydrated compared to its dry form .
Data Tables
Case Study 1: Phenol Removal from Wastewater
A manufacturer specializing in thermoset resins implemented a treatment system that successfully reduced phenol concentrations in wastewater by approximately 99%, demonstrating the efficacy of chemical treatment processes involving this compound .
Case Study 2: DNA Extraction Protocol
In a laboratory setting, a protocol utilizing this compound for DNA extraction was developed, showcasing its effectiveness in separating nucleic acids from proteins while maintaining sample integrity .
Q & A
Basic Research Questions
Q. How can researchers confirm the presence of phenol hydrate in a synthetic mixture?
Methodological Answer:
- Use Fourier-transform infrared spectroscopy (FTIR) to identify characteristic O–H stretching (~3300 cm⁻¹) and aromatic C=C vibrations (~1600 cm⁻¹).
- Compare NMR data (¹H and ¹³C) with reference spectra: phenolic protons appear as a singlet at ~5 ppm (¹H), and aromatic carbons resonate at ~115–155 ppm (¹³C).
- Validate against thermochemical databases (e.g., NIST) for molecular weight (94.11 g/mol) and enthalpy of formation (ΔfH°gas = -96.36 ± 0.59 kJ/mol) .
Q. What are the standard synthesis routes for this compound derivatives?
Methodological Answer:
- Esterification: React phenol with acetic anhydride under acidic catalysis to form phenyl acetate. Monitor reaction progress via TLC (Rf ~0.5 in hexane:ethyl acetate 3:1) .
- Electrophilic substitution: Perform nitration using HNO₃/H₂SO₄ at 50°C to yield nitrophenol isomers. Isolate para-isomer via recrystallization (mp 113–115°C) .
- Fries rearrangement: Heat phenyl acetate with AlCl₃ to produce hydroxyacetophenone derivatives. Optimize temperature (80–100°C) to minimize side products .
Q. What safety protocols are critical when handling this compound in the lab?
Methodological Answer:
- Use engineering controls (fume hoods) to maintain airborne concentrations below 5 ppm (OSHA PEL).
- Equip labs with emergency showers and eye wash stations for immediate decontamination.
- Implement PPE protocols : nitrile gloves, lab coats, and safety goggles. Train personnel on spill management (neutralize with 10% NaOH) .
Advanced Research Questions
Q. How can analytical methods resolve contradictions in this compound’s thermochemical data?
Methodological Answer:
- Re-evaluate ΔfH° discrepancies (e.g., Cox (1961): -96.36 kJ/mol vs. Parks (1954): -94.2 kJ/mol) using modern calorimetry (e.g., DSC) or computational methods (DFT).
- Cross-reference gas-phase IR spectroscopy to validate vibrational modes and refine entropy calculations .
- Publish uncertainty analyses (e.g., ±0.59 kJ/mol in Cox’s work) to contextualize historical data limitations .
Q. What advanced techniques quantify phenol hydrogenation products in complex matrices?
Methodological Answer:
- Apply headspace gas chromatography (HS-GC) with anhydrous CaCl₂ to remove water via hydrate formation, improving sensitivity for cyclohexanone/cyclohexanol detection.
- Optimize equilibrium conditions: 150°C for 5 min achieves <5.3% RSD and 93–104% recovery. Validate with spiked samples and internal standards (e.g., dodecane) .
- Compare kinetics under supercritical water (SWRS) vs. conventional hydrogenation. Monitor intermediates via in-situ Raman spectroscopy .
Q. How do substituents influence this compound’s acidity in catalytic applications?
Methodological Answer:
- Synthesize substituted derivatives (e.g., nitro-, methyl-phenol) and measure pKa via potentiometric titration (0.1 M KCl, 25°C).
- Electron-withdrawing groups (e.g., -NO₂) lower pKa (enhance acidity), while -CH₃ increases pKa. Correlate trends with Hammett σ constants .
- Use DFT calculations (B3LYP/6-311+G(d,p)) to model charge distribution and predict substituent effects on reaction pathways .
Preparation Methods
Historical Development of Phenol Hydrate Synthesis
Early attempts to synthesize this compound date to the 19th century, with Calvert (1865) initially reporting a hemihydrate form. However, reproducibility challenges plagued these efforts due to the tendency of phenol-water mixtures to supercool and crystallize as pure phenol rather than the hydrate. Breakthroughs emerged in the 20th century when rapid freezing techniques using dry ice or liquid air were employed to nucleate hydrate crystals, bypassing phase separation issues. These methods established the foundation for modern synthetic protocols, though structural ambiguities persisted until recent neutron diffraction studies resolved the crystal lattice.
Stoichiometric Considerations and Phase Behavior
The phenol-water system exhibits a narrow hydration window, with the hemihydrate (1:0.5 phenol-to-water ratio) being the most stable crystalline form under ambient conditions. Deviations from this ratio favor liquid-liquid phase separation or crystallization of anhydrous phenol. The table below summarizes critical phase behavior observations:
Table 1: Phase Behavior of Phenol-Water Mixtures
Phenol:Water Ratio | Temperature Range (°C) | Observed Outcome |
---|---|---|
1:0.5 | -20 to 25 | Stable hemihydrate crystallization |
1:1 | <0 | Metastable monohydrate (rare) |
>1:0.5 | >25 | Phase separation into layers |
The immiscibility gap necessitates rigorous stoichiometric control, as excess water or phenol disrupts hydrate nucleation.
Rapid Freezing Techniques for Hydrate Nucleation
Rapid freezing remains the most reliable method for synthesizing phenol hemihydrate. By quenching stoichiometric mixtures to temperatures below -70°C, kinetic trapping of the hydrate structure occurs before phase separation. Key steps include:
- Preparation of Stoichiometric Solution : Dissolving phenol and water in a 2:1 molar ratio under gentle heating (40–50°C) to ensure homogeneity.
- Quenching : Immersing the mixture in liquid nitrogen or dry ice/acetone baths to achieve cooling rates exceeding 100°C/min.
- Seeding : Introducing pre-formed hydrate crystals to direct crystallization, minimizing amorphous byproducts.
This method yields polycrystalline aggregates suitable for structural analysis, though single-crystal growth requires slower annealing post-quenching.
Crystallization from Aqueous Solutions
Alternative approaches involve saturating aqueous phenol solutions under controlled evaporation. However, this method is less reliable due to water’s high volatility and phenol’s low solubility. Modifications include:
- Temperature-Gradient Crystallization : Maintaining a gradient from 5°C (reservoir) to -10°C (growth zone) to slowly concentrate the solution.
- Additive-Assisted Crystallization : Incorporating trace surfactants (e.g., sodium dodecyl sulfate) to stabilize micellar structures, though this risks introducing impurities.
Table 2: Comparison of Crystallization Methods
Method | Yield (%) | Crystal Quality | Reproducibility |
---|---|---|---|
Rapid freezing | 85–90 | Polycrystalline | High |
Temperature gradient | 60–70 | Single-crystal | Moderate |
Additive-assisted | 50–60 | Amorphous | Low |
Structural Characterization and Validation
Post-synthesis validation is critical to confirming hydrate formation. Neutron powder diffraction (NPD) and X-ray diffraction (XRD) have elucidated the hemihydrate’s orthorhombic lattice (space group Pbcn), with water molecules bridging phenol dimers via O—H⋯O hydrogen bonds. Key findings include:
- Hydrogen Bonding : Each water molecule forms two donor and two acceptor bonds, creating an R₄⁴(8) hydrogen-bonded motif.
- Thermal Stability : The hydrate decomposes at 25°C, reverting to anhydrous phenol and liquid water.
Table 3: Crystallographic Data for Phenol Hemihydrate
Parameter | Value |
---|---|
Space group | Pbcn |
Unit cell dimensions | a = 12.34 Å, b = 7.89 Å, c = 10.21 Å |
Density | 1.24 g/cm³ |
Hydrogen bonds | O1—D7⋯O2 (2.76 Å) |
Challenges and Reproducibility Issues
Despite methodological advances, challenges persist:
- Supercooling : Mixtures often remain liquid below the hydrate’s freezing point, requiring seeding or agitation.
- Hygroscopicity : Anhydrous phenol rapidly absorbs atmospheric moisture, complicating stoichiometric control.
- Decomposition : The hydrate’s low thermal stability necessitates storage at sub-zero temperatures.
Recent innovations, such as microfluidic crystallization platforms, promise enhanced control over nucleation kinetics and crystal size distribution.
Recent Advances in Synthesis
Modern techniques leverage computational modeling to predict optimal crystallization conditions. Density Functional Theory (DFT) simulations have identified that incomplete hydration at the air-water interface facilitates faster nucleation, a principle exploited in spray-freezing protocols. Additionally, isotopic substitution (e.g., phenol-d₆) has enabled precise neutron scattering studies, refining synthetic pathways.
Properties
CAS No. |
144796-97-4 |
---|---|
Molecular Formula |
C6H8O2 |
Molecular Weight |
112.13 g/mol |
IUPAC Name |
phenol;hydrate |
InChI |
InChI=1S/C6H6O.H2O/c7-6-4-2-1-3-5-6;/h1-5,7H;1H2 |
InChI Key |
KSSNXJHPEFVKHY-UHFFFAOYSA-N |
SMILES |
C1=CC=C(C=C1)O.O |
Canonical SMILES |
C1=CC=C(C=C1)O.O |
Origin of Product |
United States |
Disclaimer and Information on In-Vitro Research Products
Please be aware that all articles and product information presented on BenchChem are intended solely for informational purposes. The products available for purchase on BenchChem are specifically designed for in-vitro studies, which are conducted outside of living organisms. In-vitro studies, derived from the Latin term "in glass," involve experiments performed in controlled laboratory settings using cells or tissues. It is important to note that these products are not categorized as medicines or drugs, and they have not received approval from the FDA for the prevention, treatment, or cure of any medical condition, ailment, or disease. We must emphasize that any form of bodily introduction of these products into humans or animals is strictly prohibited by law. It is essential to adhere to these guidelines to ensure compliance with legal and ethical standards in research and experimentation.