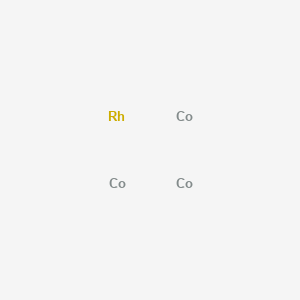
Cobalt--rhodium (3/1)
- Click on QUICK INQUIRY to receive a quote from our team of experts.
- With the quality product at a COMPETITIVE price, you can focus more on your research.
Overview
Description
Cobalt–rhodium (3/1) is a bimetallic compound composed of cobalt and rhodium in a 3:1 ratio. This compound is of significant interest due to its unique catalytic properties and potential applications in various fields, including chemistry, biology, medicine, and industry. The combination of cobalt and rhodium enhances the compound’s stability and reactivity, making it a valuable material for scientific research and industrial processes.
Preparation Methods
Synthetic Routes and Reaction Conditions
The synthesis of cobalt–rhodium (3/1) can be achieved through various methods, including chemical reduction, co-precipitation, and thermal decomposition. One common approach involves the reduction of cobalt and rhodium salts using a reducing agent such as sodium borohydride or hydrazine. The reaction is typically carried out in an aqueous or organic solvent under controlled temperature and pH conditions to ensure the formation of the desired bimetallic compound .
Industrial Production Methods
Industrial production of cobalt–rhodium (3/1) often involves large-scale chemical reduction processes. The use of high-purity cobalt and rhodium salts, along with efficient reducing agents, ensures the consistent production of the compound. Advanced techniques such as microwave-assisted synthesis and sonochemical methods have also been explored to enhance the efficiency and yield of the production process .
Chemical Reactions Analysis
Types of Reactions
Cobalt–rhodium (3/1) undergoes various chemical reactions, including oxidation, reduction, and substitution. These reactions are influenced by the compound’s unique electronic structure and the presence of both cobalt and rhodium atoms.
Common Reagents and Conditions
Oxidation: The compound can be oxidized using oxidizing agents such as hydrogen peroxide or potassium permanganate under mild conditions.
Reduction: Reduction reactions can be carried out using reducing agents like sodium borohydride or hydrazine in an aqueous or organic solvent.
Substitution: Substitution reactions often involve the replacement of ligands or functional groups on the compound using reagents such as halides or phosphines .
Major Products Formed
The major products formed from these reactions depend on the specific reagents and conditions used. For example, oxidation reactions may yield cobalt and rhodium oxides, while reduction reactions can produce metallic cobalt and rhodium nanoparticles. Substitution reactions can lead to the formation of various cobalt and rhodium complexes with different ligands .
Scientific Research Applications
Cobalt–rhodium (3/1) has a wide range of scientific research applications due to its unique catalytic properties and reactivity. Some of the key applications include:
Chemistry: The compound is used as a catalyst in various chemical reactions, including hydroformylation, hydrogenation, and C-H activation. .
Biology: In biological research, cobalt–rhodium (3/1) is explored for its potential as a therapeutic agent. .
Medicine: The compound’s catalytic properties are utilized in the development of new drugs and therapeutic agents. .
Industry: Cobalt–rhodium (3/1) is used in industrial processes such as the production of fine chemicals, pharmaceuticals, and materials. .
Mechanism of Action
The mechanism of action of cobalt–rhodium (3/1) involves its interaction with various molecular targets and pathways. In catalytic reactions, the compound facilitates the activation and transformation of substrates through its unique electronic structure and coordination environment. For example, in hydroformylation reactions, the compound activates the carbon-hydrogen bonds of olefins, leading to the formation of aldehydes .
In biological systems, rhodium complexes interact with biomolecules such as DNA, RNA, and proteins, leading to the inhibition of cellular processes and the induction of cell death. These interactions are mediated by the coordination of rhodium atoms with nucleophilic sites on the biomolecules, resulting in the disruption of their normal functions .
Comparison with Similar Compounds
Cobalt–rhodium (3/1) can be compared with other bimetallic compounds such as cobalt–palladium, cobalt–platinum, and rhodium–copper. Each of these compounds has unique properties and applications:
Cobalt–palladium: Known for its excellent catalytic activity in hydrogenation and carbon-carbon coupling reactions.
Cobalt–platinum: Exhibits high stability and catalytic efficiency in oxidation and reduction reactions.
Rhodium–copper: Demonstrates enhanced selectivity and activity in hydrogenation reactions under ambient conditions .
The uniqueness of cobalt–rhodium (3/1) lies in its ability to combine the catalytic properties of both cobalt and rhodium, resulting in a versatile and efficient catalyst for a wide range of applications.
Properties
CAS No. |
196190-47-3 |
---|---|
Molecular Formula |
Co3Rh |
Molecular Weight |
279.7051 g/mol |
IUPAC Name |
cobalt;rhodium |
InChI |
InChI=1S/3Co.Rh |
InChI Key |
KQHINJWDSMGBLD-UHFFFAOYSA-N |
Canonical SMILES |
[Co].[Co].[Co].[Rh] |
Origin of Product |
United States |
Disclaimer and Information on In-Vitro Research Products
Please be aware that all articles and product information presented on BenchChem are intended solely for informational purposes. The products available for purchase on BenchChem are specifically designed for in-vitro studies, which are conducted outside of living organisms. In-vitro studies, derived from the Latin term "in glass," involve experiments performed in controlled laboratory settings using cells or tissues. It is important to note that these products are not categorized as medicines or drugs, and they have not received approval from the FDA for the prevention, treatment, or cure of any medical condition, ailment, or disease. We must emphasize that any form of bodily introduction of these products into humans or animals is strictly prohibited by law. It is essential to adhere to these guidelines to ensure compliance with legal and ethical standards in research and experimentation.