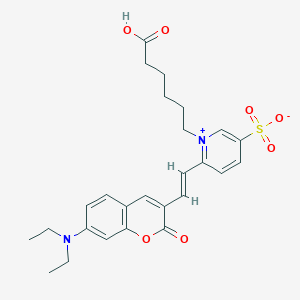
Fluorescent Red Mega 480
Overview
Description
Fluorescent Red Mega 480 is a fluorescent dye specifically designed for multicolor techniques. It is characterized by an extremely large Stoke’s shift between excitation and emission maxima, making it highly suitable for excitation by argon lasers or other short wavelength light sources . This dye can be excited using the same light source or filter settings as for fluorescein and other widely used dyes .
Biochemical Analysis
Biochemical Properties
Fluorescent Red Mega 480 plays a significant role in biochemical reactions, particularly in fluorescence-based assays. It interacts with various biomolecules, including proteins and nucleic acids, through covalent and non-covalent interactions. The dye’s small size and ability to convert excitation light directly into emission without using a FRET mechanism make it ideal for applications such as DNA sequencing and FISH microscopy . This compound can be conjugated to proteins and other biomolecules containing primary amino groups, allowing for specific labeling and detection in complex biological samples .
Cellular Effects
This compound influences various cellular processes by enabling the visualization of cellular structures and dynamics. It is used to label different cellular components, such as membranes, cytoskeleton, and organelles, allowing researchers to study cell signaling pathways, gene expression, and cellular metabolism . The dye’s ability to be excited by the same light source as fluorescein and other widely used dyes makes it versatile for multicolor imaging experiments .
Molecular Mechanism
At the molecular level, this compound exerts its effects through specific binding interactions with biomolecules. The dye’s NHS ester form allows for covalent coupling to proteins and other biomolecules containing primary amino groups . This covalent attachment ensures stable labeling and minimizes the risk of dye dissociation during experiments. Additionally, the dye’s large stoke’s shift between excitation and emission maxima enhances its sensitivity and reduces background fluorescence .
Temporal Effects in Laboratory Settings
In laboratory settings, the effects of this compound can change over time due to factors such as stability and degradation. The dye is generally stable when stored at recommended conditions (2-8°C) and protected from light . Prolonged exposure to light or suboptimal storage conditions can lead to degradation and reduced fluorescence intensity. Long-term studies have shown that the dye maintains its fluorescence properties over extended periods, making it suitable for both short-term and long-term experiments .
Dosage Effects in Animal Models
The effects of this compound vary with different dosages in animal models. At low doses, the dye provides clear and specific labeling of target biomolecules without causing significant toxicity . At high doses, there may be threshold effects, including potential toxicity and adverse effects on cellular function. It is essential to optimize the dosage to achieve the desired labeling while minimizing any potential negative impacts .
Metabolic Pathways
This compound is involved in various metabolic pathways, particularly those related to fluorescence-based detection and imaging. The dye interacts with enzymes and cofactors that facilitate its incorporation into biological samples . These interactions can affect metabolic flux and metabolite levels, providing valuable insights into cellular metabolism and biochemical processes .
Transport and Distribution
Within cells and tissues, this compound is transported and distributed through specific transporters and binding proteins . These interactions influence the dye’s localization and accumulation in different cellular compartments. The dye’s small size and ability to bind to primary amino groups allow for efficient transport and distribution, ensuring accurate labeling of target biomolecules .
Subcellular Localization
This compound exhibits specific subcellular localization, which can affect its activity and function. The dye is often directed to specific compartments or organelles through targeting signals or post-translational modifications . This precise localization enables researchers to study the dynamics and interactions of biomolecules within distinct cellular regions, providing valuable insights into cellular function and organization .
Preparation Methods
The synthetic routes and reaction conditions for Fluorescent Red Mega 480 involve the use of various organic synthesis techniques. The compound is typically prepared through a series of reactions that introduce the necessary functional groups to achieve the desired fluorescence properties. Industrial production methods focus on optimizing yield and purity, often involving high-performance liquid chromatography (HPLC) to ensure the product meets the required specifications .
Chemical Reactions Analysis
Fluorescent Red Mega 480 undergoes several types of chemical reactions, including:
Oxidation: The compound can be oxidized under specific conditions, leading to changes in its fluorescence properties.
Reduction: Reduction reactions can also alter the fluorescence characteristics of the dye.
Substitution: The dye can undergo substitution reactions where functional groups are replaced with others, potentially modifying its fluorescence behavior.
Common reagents and conditions used in these reactions include strong oxidizing agents, reducing agents, and various catalysts. The major products formed from these reactions depend on the specific reagents and conditions used .
Scientific Research Applications
High-Throughput Screening (HTS)
Fluorescent Red Mega 480 is extensively utilized in high-throughput screening assays due to its sensitivity and robustness. The dye's ability to report on molecular interactions, such as protein-protein or protein-nucleic acid interactions, makes it ideal for drug discovery.
- Case Study : A recent study utilized this compound in an HTS assay to identify inhibitors of the interaction between human antigen R (HuR) and RNA. The assay demonstrated high sensitivity and specificity, allowing for the screening of thousands of compounds effectively .
Flow Cytometry
The dye is compatible with flow cytometry applications, allowing for the analysis of multiple fluorescent markers simultaneously. Its low autofluorescence and efficient excitation properties enhance the accuracy of cell sorting and analysis.
- Case Study : In a comparative analysis of various fluorescent proteins, this compound was incorporated into multi-color flow cytometry experiments, demonstrating superior performance in reducing background fluorescence compared to traditional dyes .
Imaging Techniques
This compound is also employed in imaging applications, particularly in live cell imaging where real-time observation of cellular processes is required. Its large Stokes shift minimizes spectral overlap with other fluorescent markers.
- Case Study : Researchers used this compound in a live-cell imaging study to track the dynamics of protein localization within cells. The results indicated that the dye provided clear visualization without significant photobleaching over extended observation periods .
Data Tables
Mechanism of Action
Fluorescent Red Mega 480 operates through the process of fluorescence. It absorbs light at a specific wavelength and subsequently emits light at a different wavelength. This direct conversion of excitation light into emission without using a Förster Resonance Energy Transfer (FRET) mechanism allows for its diverse applications in scientific research . The molecular targets and pathways involved include various cellular components that interact with the dye, facilitating its use in detecting and quantifying biological processes .
Comparison with Similar Compounds
Fluorescent Red Mega 480 is unique due to its extremely large Stoke’s shift and suitability for excitation by argon lasers. Similar compounds include:
Fluorescein: Another widely used fluorescent dye with different excitation and emission properties.
Rhodamine: A fluorescent dye with distinct spectral characteristics compared to this compound.
Cy3 and Cy5: Fluorescent dyes used in various biological applications, each with unique excitation and emission wavelengths.
This compound stands out due to its ability to be excited using the same light source or filter settings as for fluorescein and other widely used dyes, making it highly versatile for multicolor techniques .
Biological Activity
Fluorescent Red Mega 480 is a synthetic dye renowned for its vibrant red fluorescence and significant applications in biological research, particularly in imaging and labeling techniques. This article delves into its biological activity, photophysical properties, and practical applications, supported by data tables and case studies.
Overview of this compound
This compound is characterized by a large Stokes shift, which enhances its utility in multicolor fluorescence applications. The compound's chemical structure features a complex arrangement of aromatic rings that contribute to its strong photophysical properties, including high quantum yield and stability under various conditions .
Key Properties
- Emission Wavelength : 480 nm
- Stokes Shift : Large
- Quantum Yield : High
- Stability : Excellent under varying experimental conditions
This compound interacts with biomolecules primarily through non-covalent interactions such as hydrogen bonding and van der Waals forces. This interaction enables the dye to effectively label cellular structures and processes, making it a valuable tool for live-cell imaging .
Applications in Imaging
The dye's strong fluorescence allows for effective visualization of cellular events. It has been employed in various biological applications, including:
- Fluorescence Microscopy : Used to track cellular dynamics in live cells.
- Flow Cytometry : Facilitates the analysis of cell populations based on fluorescence characteristics.
- Medical Diagnostics : Enhances the visualization of biomarkers in clinical samples.
Comparative Analysis with Other Dyes
The following table compares this compound with other common fluorescent dyes:
Compound Name | Emission Wavelength | Stokes Shift | Unique Features |
---|---|---|---|
This compound | 480 nm | Large | High quantum yield; strong interaction with nanoparticles |
Rhodamine B | 570 nm | Moderate | Commonly used but less stable under light |
Texas Red | 615 nm | Moderate | Excellent for long-range imaging |
Cy3 | 550 nm | Small | Often used in nucleic acid labeling |
This compound stands out due to its superior stability and large Stokes shift, making it particularly advantageous for multicolor applications where overlapping emission spectra could complicate analysis.
High-Throughput Screening
A study conducted at the National Center for Advancing Translational Sciences (NCATS) utilized this compound in high-throughput fluorescence polarization assays. The research demonstrated the dye's effectiveness in detecting molecular interactions within complex biological systems. The results indicated that the dye could provide reliable readouts in screening environments, enhancing the throughput of drug discovery processes .
Cellular Imaging
Another significant application involved tracking nitrogenase gene expression in photosynthetic bacteria using a fluorescent protein reporter system based on this compound. The study showed that fluorescence intensity increased significantly under specific conditions, confirming the dye's utility in monitoring gene expression dynamically within living cells .
Properties
IUPAC Name |
1-(5-carboxypentyl)-6-[(E)-2-[7-(diethylamino)-2-oxochromen-3-yl]ethenyl]pyridin-1-ium-3-sulfonate | |
---|---|---|
Source | PubChem | |
URL | https://pubchem.ncbi.nlm.nih.gov | |
Description | Data deposited in or computed by PubChem | |
InChI |
InChI=1S/C26H30N2O7S/c1-3-27(4-2)22-12-9-19-16-20(26(31)35-24(19)17-22)10-11-21-13-14-23(36(32,33)34)18-28(21)15-7-5-6-8-25(29)30/h9-14,16-18H,3-8,15H2,1-2H3,(H-,29,30,32,33,34) | |
Source | PubChem | |
URL | https://pubchem.ncbi.nlm.nih.gov | |
Description | Data deposited in or computed by PubChem | |
InChI Key |
XWKHECGJHWMWTB-UHFFFAOYSA-N | |
Source | PubChem | |
URL | https://pubchem.ncbi.nlm.nih.gov | |
Description | Data deposited in or computed by PubChem | |
Canonical SMILES |
CCN(CC)C1=CC2=C(C=C1)C=C(C(=O)O2)C=CC3=[N+](C=C(C=C3)S(=O)(=O)[O-])CCCCCC(=O)O | |
Source | PubChem | |
URL | https://pubchem.ncbi.nlm.nih.gov | |
Description | Data deposited in or computed by PubChem | |
Isomeric SMILES |
CCN(CC)C1=CC2=C(C=C1)C=C(C(=O)O2)/C=C/C3=[N+](C=C(C=C3)S(=O)(=O)[O-])CCCCCC(=O)O | |
Source | PubChem | |
URL | https://pubchem.ncbi.nlm.nih.gov | |
Description | Data deposited in or computed by PubChem | |
Molecular Formula |
C26H30N2O7S | |
Source | PubChem | |
URL | https://pubchem.ncbi.nlm.nih.gov | |
Description | Data deposited in or computed by PubChem | |
DSSTOX Substance ID |
DTXSID60583667 | |
Record name | 1-(5-Carboxypentyl)-6-{(E)-2-[7-(diethylamino)-2-oxo-2H-1-benzopyran-3-yl]ethenyl}pyridin-1-ium-3-sulfonate | |
Source | EPA DSSTox | |
URL | https://comptox.epa.gov/dashboard/DTXSID60583667 | |
Description | DSSTox provides a high quality public chemistry resource for supporting improved predictive toxicology. | |
Molecular Weight |
514.6 g/mol | |
Source | PubChem | |
URL | https://pubchem.ncbi.nlm.nih.gov | |
Description | Data deposited in or computed by PubChem | |
CAS No. |
540528-00-5 | |
Record name | 1-(5-Carboxypentyl)-6-{(E)-2-[7-(diethylamino)-2-oxo-2H-1-benzopyran-3-yl]ethenyl}pyridin-1-ium-3-sulfonate | |
Source | EPA DSSTox | |
URL | https://comptox.epa.gov/dashboard/DTXSID60583667 | |
Description | DSSTox provides a high quality public chemistry resource for supporting improved predictive toxicology. | |
Retrosynthesis Analysis
AI-Powered Synthesis Planning: Our tool employs the Template_relevance Pistachio, Template_relevance Bkms_metabolic, Template_relevance Pistachio_ringbreaker, Template_relevance Reaxys, Template_relevance Reaxys_biocatalysis model, leveraging a vast database of chemical reactions to predict feasible synthetic routes.
One-Step Synthesis Focus: Specifically designed for one-step synthesis, it provides concise and direct routes for your target compounds, streamlining the synthesis process.
Accurate Predictions: Utilizing the extensive PISTACHIO, BKMS_METABOLIC, PISTACHIO_RINGBREAKER, REAXYS, REAXYS_BIOCATALYSIS database, our tool offers high-accuracy predictions, reflecting the latest in chemical research and data.
Strategy Settings
Precursor scoring | Relevance Heuristic |
---|---|
Min. plausibility | 0.01 |
Model | Template_relevance |
Template Set | Pistachio/Bkms_metabolic/Pistachio_ringbreaker/Reaxys/Reaxys_biocatalysis |
Top-N result to add to graph | 6 |
Feasible Synthetic Routes
Q1: What are the key spectroscopic properties of Red Mega 480 in solution?
A1: While the provided research abstract does not specify the exact molecular formula, weight, or complete spectroscopic data for Red Mega 480, it highlights that the study investigates the compound's fluorescence properties in various solutions. [] This suggests the research likely explored aspects like absorption and emission spectra, fluorescence quantum yields, and fluorescence lifetimes of Red Mega 480 in different solvents. This information is crucial for understanding its potential applications, especially as a laser dye.
Disclaimer and Information on In-Vitro Research Products
Please be aware that all articles and product information presented on BenchChem are intended solely for informational purposes. The products available for purchase on BenchChem are specifically designed for in-vitro studies, which are conducted outside of living organisms. In-vitro studies, derived from the Latin term "in glass," involve experiments performed in controlled laboratory settings using cells or tissues. It is important to note that these products are not categorized as medicines or drugs, and they have not received approval from the FDA for the prevention, treatment, or cure of any medical condition, ailment, or disease. We must emphasize that any form of bodily introduction of these products into humans or animals is strictly prohibited by law. It is essential to adhere to these guidelines to ensure compliance with legal and ethical standards in research and experimentation.