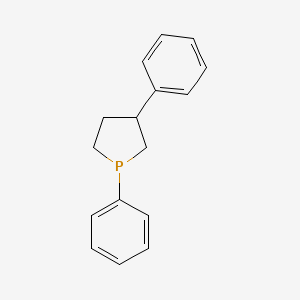
Phospholane, 1,3-diphenyl-
- Click on QUICK INQUIRY to receive a quote from our team of experts.
- With the quality product at a COMPETITIVE price, you can focus more on your research.
Overview
Description
1,3-Diphenylphospholane is a five-membered saturated heterocyclic compound containing one phosphorus atom and four carbon atoms in the ring, with phenyl substituents at the 1- and 3-positions . Classified under phospholanes, this compound exhibits a unique electronic environment due to the phosphorus atom, which can adopt varying oxidation states and participate in coordination chemistry. The phenyl groups contribute steric bulk and electronic effects, influencing its reactivity and applications in catalysis or ligand design. Phospholanes are structurally distinct from other heterocycles due to the presence of phosphorus, which imparts distinct Lewis basicity and redox properties compared to nitrogen- or oxygen-containing analogs .
Preparation Methods
Synthetic Routes and Reaction Conditions: Phospholane, 1,3-diphenyl- can be synthesized through several methods. One common approach involves the reaction of chlorophosphines with Grignard reagents. For instance, the interaction of chlorophosphines with phenylmagnesium bromide can yield phospholane derivatives . Another method involves the use of organolithium reagents in the presence of suitable catalysts .
Industrial Production Methods: Industrial production of phospholane, 1,3-diphenyl- typically involves large-scale reactions using similar synthetic routes as mentioned above. The choice of reagents and conditions is optimized to ensure high yield and purity of the final product. The use of continuous flow reactors and automated systems can enhance the efficiency and scalability of the production process.
Chemical Reactions Analysis
Types of Reactions: Phospholane, 1,3-diphenyl- undergoes various chemical reactions, including oxidation, reduction, and substitution reactions.
Common Reagents and Conditions:
Substitution: Substitution reactions can occur at the phosphorus atom or the phenyl rings, depending on the reagents and conditions used.
Major Products: The major products formed from these reactions include phosphine oxides, reduced phosphines, and substituted phospholane derivatives.
Scientific Research Applications
Hydroformylation Catalysis
Phospholane-based ligands have been shown to exhibit significant selectivity in hydroformylation reactions, where terminal alkenes are converted into aldehydes. Research indicates that phospholane-phosphites can selectively produce branched aldehydes, enhancing the efficiency of this reaction. For example, a study demonstrated that a specific phospholane ligand achieved a branched selectivity of 67.5% for iso-butanal at elevated temperatures, showcasing its potential in industrial applications .
Table 1: Hydroformylation Selectivity Data
Ligand Type | Product | Selectivity (%) | Temperature (°C) |
---|---|---|---|
Phospholane-phosphite | Iso-butanal | 67.5 | 75 |
Unsubstituted ligand | Iso-butanal | 74.6 | 75 |
Organocatalysis
Phosphorus-based organocatalysts derived from Phospholane, 1,3-diphenyl- have gained traction due to their ability to facilitate various organic transformations. These catalysts are characterized by their high reactivity and selectivity in reactions such as Michael additions and cyclizations . The introduction of bulky groups in phospholane derivatives can enhance catalytic activity while maintaining selectivity.
Anticancer Activity
Recent studies have highlighted the cytotoxic effects of compounds related to Phospholane, 1,3-diphenyl- against cancer cells. For instance, research involving synthesized derivatives demonstrated significant cytotoxicity against breast cancer cell lines (MCF-7), while exhibiting low toxicity towards normal cells . This dual activity makes these compounds promising candidates for further development as anticancer agents.
Table 2: Cytotoxicity of Phospholane Derivatives
Compound Type | Cell Line | Cytotoxicity (IC50 µM) | Normal Cell Toxicity |
---|---|---|---|
Phospholane derivative | MCF-7 | 15 | Low |
Control (Tamoxifen) | MCF-7 | 10 | Moderate |
Synthesis Techniques
Phospholane derivatives can be synthesized via various methods, including one-pot reactions that yield diastereoisomeric products with high efficiency . The synthesis involves using diphenyl phosphine oxide as a key starting material, which reacts under mild conditions to form the desired phospholane structure.
Characterization Methods
Characterization of Phospholane compounds typically employs techniques such as NMR spectroscopy and mass spectrometry to confirm molecular structure and purity. For example, 31P NMR provides insights into the electronic environment of phosphorus atoms within the phospholane ring .
Industrial Applications
A case study on the use of Phospholane-based ligands in commercial hydroformylation processes demonstrated an increase in yield and selectivity when utilizing these specialized ligands compared to traditional catalysts. This improvement not only enhances product quality but also reduces waste and costs associated with catalyst recovery.
Academic Research
Another case study focused on the development of new phosphine-phospholene ligands for asymmetric synthesis revealed that these ligands significantly improve reaction rates and selectivities in various transformations, indicating their versatility and importance in synthetic organic chemistry .
Mechanism of Action
The mechanism by which phospholane, 1,3-diphenyl- exerts its effects depends on the specific reaction or application. In catalytic processes, the compound acts as a ligand, coordinating with metal centers to facilitate various chemical transformations . The molecular targets and pathways involved can vary widely, but typically involve interactions with electrophilic or nucleophilic sites on the substrate molecules.
Comparison with Similar Compounds
Structural and Electronic Comparisons
1,3,2-Dioxaphospholane-2-oxide (CAS 1003-11-8)
This compound features a five-membered ring with one phosphorus and two oxygen atoms. Unlike 1,3-diphenylphospholane, the oxygen atoms increase ring polarity and alter the electronic environment. The oxide group on phosphorus enhances electrophilicity, making it more reactive toward nucleophiles. Applications differ significantly: 1,3,2-dioxaphospholane derivatives are often intermediates in organophosphorus synthesis, whereas 1,3-diphenylphospholane may serve as a ligand in asymmetric catalysis due to its steric profile .
1,3-Diphenyl Triazene (CAS not provided)
A linear triazene (N–N=N–) backbone substituted with phenyl groups, this compound lacks a heterocyclic ring but shares aryl substituents. Triazenes are redox-active and thermally unstable compared to phospholanes, often decomposing to release nitrogen. They are utilized in pharmaceuticals (e.g., temozolomide analogs) and as diazo precursors, contrasting with phospholanes’ roles in coordination chemistry .
1,3-Dioxolane Derivatives (e.g., CAS 89303-50-4)
These oxygen-containing heterocycles lack phosphorus but share a five-membered ring structure. The nitro and sulfonyl substituents in CAS 89303-50-4 create strong electron-withdrawing effects, rendering the compound more electrophilic than 1,3-diphenylphospholane. Such dioxolanes are often used in polymer chemistry or as solvents, diverging from phospholanes’ catalytic applications .
Physicochemical Properties and Reactivity
Property | 1,3-Diphenylphospholane | 1,3,2-Dioxaphospholane-2-oxide | 1,3-Diphenyl Triazene | 1,3-Dioxolane (CAS 89303-50-4) |
---|---|---|---|---|
Heteroatoms | P, C₄ | P, O₂, C₂ | N₃, C₆H₅ | O₂, C₃, NO₂, SO₂ |
Key Substituents | Phenyl (1,3-) | Oxide (P=O) | Phenyl (1,3-) | Nitro, sulfonyl |
Polarity | Moderate (P-centered Lewis base) | High (P=O dipole) | Low (nonpolar aryl groups) | High (EWG substituents) |
Thermal Stability | High (saturated ring) | Moderate (oxide prone to hydrolysis) | Low (N–N bonds labile) | Moderate (stable under anhydrous) |
Primary Applications | Catalysis, ligands | Organophosphorus synthesis | Pharmaceuticals, diazo chemistry | Polymers, specialty solvents |
Spectroscopic Characterization
1,3-Diphenylphospholane can be analyzed via ¹H and ¹³C NMR, similar to triazenes and dioxolanes. However, the phosphorus atom enables ³¹P NMR, a tool absent in nitrogen/oxygen analogs. For example, ¹H NMR of triazenes (e.g., 1,3-diphenyl triazene) shows distinct N–H signals, while phospholanes exhibit coupling between P and adjacent protons .
Biological Activity
Phospholane, 1,3-diphenyl- is a compound of interest due to its potential biological activities. This article explores the synthesis, biological evaluations, and mechanisms of action associated with this compound, drawing from diverse research findings.
Phospholane compounds are often synthesized through various catalytic processes. A notable method involves using diphenylphosphinic acid as an efficient organocatalyst in multi-component reactions. The resulting phosphonates exhibit significant yields and purity, which are essential for biological testing. For instance, a study reported the synthesis of various phosphonates with high yields, indicating that phospholane derivatives can be effectively produced for further biological evaluation .
Biological Activity Overview
Anticancer Properties
Research has indicated that 1,3-diphenyl-1H-pyrazole derivatives exhibit notable anticancer properties. These compounds have been shown to induce apoptosis in cancer cells by collapsing mitochondrial membrane potential and increasing reactive oxygen species (ROS) levels. Such mechanisms suggest that phospholane derivatives might be promising candidates for cancer therapeutics .
Antioxidant Activity
In vitro studies have demonstrated that certain phospholane derivatives possess antioxidant properties, outperforming traditional antioxidants like ascorbic acid (Vitamin C). This activity is crucial for protecting cells from oxidative stress and could have implications in preventing cellular damage associated with various diseases .
Case Study: Anticancer Activity
A specific study evaluated the biological activity of 1,3-diphenyl-1H-pyrazole derivatives against various cancer cell lines. The results indicated:
- IC50 Values : The compounds showed IC50 values ranging from 10 to 30 µM, indicating potent anti-proliferative effects.
- Mechanism of Action : The induction of apoptosis was confirmed through flow cytometry and Western blot analysis, showing increased levels of pro-apoptotic markers and decreased levels of anti-apoptotic proteins.
Table 1: Summary of Biological Activities
Compound | Activity Type | IC50 (µM) | Mechanism of Action |
---|---|---|---|
1,3-Diphenyl-1H-pyrazole | Anticancer | 10-30 | Induces apoptosis via ROS generation |
Diphenylphosphinic Acid | Antioxidant | <50 | Scavenges free radicals |
Phosphonate Derivative | Urease Inhibition | 15-30 | Mixed-type inhibition with significant binding interactions |
Mechanisms Underlying Biological Activities
The biological activities associated with phospholane derivatives can be attributed to their chemical structure and reactivity:
- Reactive Oxygen Species (ROS) Generation : The ability to generate ROS is a critical factor in the anticancer activity of these compounds. Elevated ROS levels can lead to oxidative stress in cancer cells, triggering apoptosis.
- Enzyme Inhibition : Some phospholane derivatives have been studied for their potential to inhibit urease activity, which is relevant in treating conditions like kidney stones and certain infections. The IC50 values indicate significant inhibitory effects against urease enzymes .
Q & A
Q. What are the established synthetic routes for 1,3-diphenylphospholane, and how do reaction conditions influence yield and purity?
Basic Research Question
The synthesis of 1,3-diphenylphospholane typically involves cyclization reactions using phosphorus precursors. A common method (Scheme 4.3 in ) employs N,N-diethylphospholan-1-amine as an intermediate, generated via reactions between 1,4-bis(bromomagnesio)butane and dichlorophosphines. Reaction conditions such as temperature (−78°C to room temperature), solvent polarity (diethyl ether or THF), and stoichiometric control of Grignard reagents critically affect yield and purity. For example, excess Grignard reagent can lead to side products like lithium phospholane derivatives (Scheme 4.5, ). Purification via column chromatography or recrystallization is essential to isolate the target compound .
Q. What spectroscopic and crystallographic techniques are most effective for characterizing 1,3-diphenylphospholane derivatives?
Basic Research Question
Key techniques include:
- 31P NMR spectroscopy : Identifies phosphorus environments and ligand coordination shifts (e.g., δ ~20–30 ppm for free phospholanes vs. δ −10–10 ppm for metal-bound species) .
- X-ray crystallography : Resolves stereoelectronic effects, as shown in Figure 4.2 ( ), where the Fe–P bond length (2.25 Å) confirms ligand-metal interactions.
- UV-Vis spectroscopy : Monitors ligand substitution in catalytic applications (e.g., absorbance shifts at 418 nm in singlet oxygen trapping experiments, as in ).
Q. What safety protocols are critical when handling 1,3-diphenylphospholane derivatives, particularly regarding toxicity and environmental hazards?
Basic Research Question
- Toxicity : Use fume hoods and PPE to avoid inhalation or skin contact, as phospholanes may act as irritants ( ).
- Environmental hazards : Prevent groundwater contamination via sealed waste containers. Compounds like 1,3,2-dioxaphospholane derivatives ( ) require neutralization before disposal due to hydrolytic release of HCl.
- Storage : Store under inert gas (N2/Ar) at −20°C to prevent oxidation .
Q. What statistical approaches are recommended for analyzing discrepancies in ligand geometry predictions versus experimental crystallographic data?
Advanced Research Question
- Root-mean-square deviation (RMSD) analysis : Quantifies differences between DFT-optimized structures (e.g., bond angles, torsion angles) and X-ray data.
- Multivariate regression : Correlates steric/electronic parameters (Tolman cone angles, natural bond orbital charges) with experimental deviations .
- Error-weighted refinement : Adjusts computational models using crystallographic residual factors (Rfree) to improve predictive accuracy .
Q. How can researchers resolve contradictions in reported catalytic activities of 1,3-diphenylphospholane-based complexes across different studies?
Advanced Research Question
Contradictions often arise from:
- Ligand lability : Phospholanes may dissociate under high-temperature catalysis, altering active species ( ).
- Solvent effects : Polar solvents (e.g., DMF) stabilize ionic intermediates, while nonpolar solvents favor neutral pathways.
- Methodological rigor : Standardize turnover number (TON) calculations by accounting for trace moisture or oxygen ( ). Cross-validate using in situ IR or EPR to track reaction intermediates .
Q. What methodologies assess the environmental impact of 1,3-diphenylphospholane synthesis byproducts?
Advanced Research Question
- Life-cycle assessment (LCA) : Quantify waste streams (e.g., chlorinated byproducts in ) using tools like ECHA’s SCIP database.
- Ecotoxicology assays : Test acute toxicity (LC50) of synthesis residues on Daphnia magna or algal models ().
- Green chemistry metrics : Calculate atom economy (ideal: 100%) and E-factor (kg waste/kg product) for solvent-intensive steps .
Q. How can DFT calculations guide the design of 1,3-diphenylphospholane ligands for CO2_22 hydrogenation catalysts?
Advanced Research Question
- Electronic structure modeling : Compute frontier molecular orbitals (HOMO/LUMO) to predict ligand-metal charge transfer (e.g., Fe–CO2 binding energies in ).
- Transition state analysis : Identify rate-limiting steps (e.g., H2 heterolytic cleavage) using nudged elastic band (NEB) methods.
- Steric maps : Generate %Vbur values to optimize ligand bulkiness for substrate access .
Properties
CAS No. |
590374-93-9 |
---|---|
Molecular Formula |
C16H17P |
Molecular Weight |
240.28 g/mol |
IUPAC Name |
1,3-diphenylphospholane |
InChI |
InChI=1S/C16H17P/c1-3-7-14(8-4-1)15-11-12-17(13-15)16-9-5-2-6-10-16/h1-10,15H,11-13H2 |
InChI Key |
NPGQUSIJDKYQTK-UHFFFAOYSA-N |
Canonical SMILES |
C1CP(CC1C2=CC=CC=C2)C3=CC=CC=C3 |
Origin of Product |
United States |
Disclaimer and Information on In-Vitro Research Products
Please be aware that all articles and product information presented on BenchChem are intended solely for informational purposes. The products available for purchase on BenchChem are specifically designed for in-vitro studies, which are conducted outside of living organisms. In-vitro studies, derived from the Latin term "in glass," involve experiments performed in controlled laboratory settings using cells or tissues. It is important to note that these products are not categorized as medicines or drugs, and they have not received approval from the FDA for the prevention, treatment, or cure of any medical condition, ailment, or disease. We must emphasize that any form of bodily introduction of these products into humans or animals is strictly prohibited by law. It is essential to adhere to these guidelines to ensure compliance with legal and ethical standards in research and experimentation.