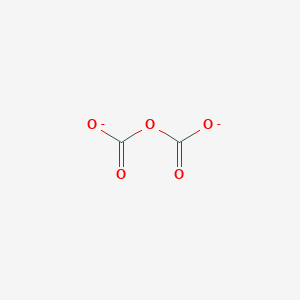
Dicarbonate
- Click on QUICK INQUIRY to receive a quote from our team of experts.
- With the quality product at a COMPETITIVE price, you can focus more on your research.
Overview
Description
Dicarbonate, also known as this compound, is a useful research compound. Its molecular formula is C2O5-2 and its molecular weight is 104.02 g/mol. The purity is usually 95%.
BenchChem offers high-quality this compound suitable for many research applications. Different packaging options are available to accommodate customers' requirements. Please inquire for more information about this compound including the price, delivery time, and more detailed information at info@benchchem.com.
Scientific Research Applications
Antimicrobial Applications
Dimethyl Dicarbonate as a Food Additive
Dimethyl this compound is primarily recognized for its effectiveness as an antimicrobial agent in the food and beverage industry. It is widely used to inhibit the growth of spoilage microorganisms in beverages such as wines and juices. The compound works by penetrating microbial cells and deactivating enzymes, which leads to cell death without altering the taste or appearance of the product .
Case Study: Efficacy Against Microorganisms
A study demonstrated that DMDC effectively inhibited various microorganisms at concentrations ranging from 0 to 250 ppm. The kinetic analysis revealed that the inhibition followed a first-order kinetic model, indicating a strong correlation between concentration and microbial reduction . Table 1 summarizes the effectiveness of DMDC against specific pathogens:
Microorganism | Concentration (ppm) | Inhibition Rate (%) |
---|---|---|
E. coli | 250 | 98 |
Yeast | 250 | 95 |
Molds | 250 | 93 |
Application in Juice Fermentation
DMDC has been shown to be a viable alternative to traditional heat treatments in juice fermentation processes. A study on litchi juice indicated that DMDC treatment preserved sensory qualities and nutritional content better than heat treatment while ensuring microbial safety . This highlights its role in maintaining product quality during fermentation.
Food Preservation
Dicarbonates like DMDC are crucial in extending the shelf life of various food products by preventing microbial spoilage. They are particularly beneficial in non-alcoholic beverages, where they stabilize carbonated drinks and flavored waters without compromising quality .
Comparative Analysis of Preservation Methods
Table 2 compares DMDC with traditional preservation methods:
Preservation Method | Advantages | Disadvantages |
---|---|---|
Dimethyl this compound | Non-altering taste, effective against a broad range of microbes | Regulatory approvals required |
Heat Treatment | Widely accepted, effective | Can alter flavor and nutritional value |
Chemical Preservatives | Cost-effective | Potential health risks |
Electrochemical Applications
Dicarbonates are also being explored for their potential in electrochemical applications, particularly as solvents or electrolytes in batteries. A recent study highlighted the use of this compound-based electrolytes that enhance battery performance by improving capacity retention and stability . The unique properties of dicarbonates allow for better formation of solid-electrolyte interphases, which are critical for battery efficiency.
Performance Metrics
The performance of this compound-based electrolytes was evaluated through various electrochemical tests. Results indicated significant improvements over conventional electrolytes:
Electrolyte Type | Capacity Retention (%) | Cycle Stability (Cycles) |
---|---|---|
Conventional Carbonates | 80 | 500 |
This compound-Based | 90 | 800 |
Q & A
Basic Research Questions
Q. What are the key safety protocols for handling Di-tert-butyl dicarbonate in laboratory settings?
Di-tert-butyl this compound is highly flammable and toxic, requiring strict safety measures. Use fume hoods to avoid inhalation, wear nitrile gloves and chemical-resistant goggles, and avoid contact with oxidizers or acids. In case of spills, evacuate the area, neutralize with inert absorbents (e.g., sand), and dispose of waste as hazardous material . Always consult SDS documents for specific storage conditions (e.g., sealed containers at <25°C) and emergency procedures.
Q. Which spectroscopic techniques are most effective for characterizing dicarbonates?
Nuclear magnetic resonance (NMR) spectroscopy (¹³C and ¹H) is critical for structural elucidation, particularly for verifying tert-butoxycarbonyl (Boc) groups. Fourier-transform infrared (FTIR) spectroscopy identifies carbonyl (C=O) stretches (~1750–1850 cm⁻¹). Mass spectrometry (MS) confirms molecular weight and fragmentation patterns, while gas chromatography (GC) or high-performance liquid chromatography (HPLC) assesses purity .
Q. How can researchers mitigate decomposition risks during this compound synthesis?
Dicarbonates degrade under heat, moisture, or acidic/basic conditions. Maintain anhydrous environments using molecular sieves or inert gas (N₂/Ar). Monitor reaction temperatures (e.g., Di-tert-butyl this compound’s boiling point: 56–57°C) to avoid thermal decomposition. Pre-purify solvents (e.g., toluene, THF) to remove traces of water or reactive impurities .
Advanced Research Questions
Q. What role do dicarbonates play in lithium-ion battery electrolyte stability?
Linear dialkyl carbonates (e.g., dimethyl carbonate) act as co-solvents in lithium-ion electrolytes, improving ionic conductivity and electrode passivation. However, dicarbonates like ethylene carbonate (EC) form solid-electrolyte interphases (SEIs) on anodes, which prevent further electrolyte decomposition. Advanced studies use cyclic voltammetry (CV) and impedance spectroscopy to quantify SEI formation efficiency and correlate it with battery cycle life .
Q. How can computational modeling predict this compound reactivity in organic synthesis?
Density functional theory (DFT) calculates activation energies for Boc protection/deprotection reactions, identifying transition states and intermediates. Molecular dynamics (MD) simulations model solvent effects on reaction kinetics. Validate models experimentally using kinetic isotope effects (KIEs) or isotopic labeling .
Q. What methodologies resolve contradictions in this compound toxicity data across studies?
Conflicting toxicity reports (e.g., LD50 variations) may arise from differences in exposure routes (inhalation vs. dermal) or model organisms. Conduct meta-analyses with standardized protocols:
- Use in vitro assays (e.g., MTT for cytotoxicity) alongside in vivo models.
- Apply Hill’s criteria for causality (e.g., dose-response consistency).
- Cross-reference with ecotoxicological databases for environmental impact .
Q. Methodological Design
Q. How to design experiments assessing this compound stability under varying pH conditions?
- Prepare buffered solutions (pH 1–14) and incubate dicarbonates at controlled temperatures (25–60°C).
- Monitor degradation via HPLC-MS at timed intervals.
- Use Arrhenius plots to derive activation energies and predict shelf-life .
Q. What statistical approaches analyze this compound-related environmental data?
Multivariate analysis (e.g., PCA) identifies correlations between this compound concentrations and ecological variables (e.g., soil pH, microbial activity). Survival analysis models ecotoxicity thresholds for aquatic organisms .
Q. Data Interpretation
Q. How to address discrepancies in spectroscopic data for novel this compound derivatives?
Contradictions in NMR/IR spectra may stem from tautomerism or solvent polarity effects. Compare data across solvents (DMSO vs. CDCl₃) and use 2D NMR (COSY, HSQC) to resolve structural ambiguities .
Q. What frameworks evaluate this compound applications in pharmaceutical intermediates?
Apply Quality by Design (QbD) principles:
Preparation Methods
Synthesis of Dimethyl Dicarbonate
Chloroformate Carbonylation
The most widely documented method for synthesizing dimethyl this compound involves the reaction of methyl chloroformate with sodium carbonate in the presence of a phase-transfer catalyst. According to CN102219690A, this process utilizes methylene chloride as a solvent and tetrabutylammonium bromide (TBAB) as a catalyst . The reaction proceeds via nucleophilic acyl substitution, where the carbonate ion attacks the electrophilic carbonyl carbon of methyl chloroformate.
Reaction Conditions:
-
Molar Ratio: Methyl chloroformate to sodium carbonate is maintained at 2:1 to ensure complete conversion.
-
Temperature: The reaction is conducted at 0–10°C to minimize side reactions such as hydrolysis.
-
Catalyst Loading: TBAB is used at 2–5 mol% relative to sodium carbonate, enhancing interfacial reactivity .
Workup and Yield:
Post-reaction, the organic layer is washed with water to remove residual salts and concentrated under reduced pressure. The patent reports yields of 89–90% with a purity exceeding 98% .
Solvent and Catalyst Optimization
The choice of solvent significantly impacts reaction efficiency. Polar aprotic solvents like methylene chloride facilitate better solubility of ionic intermediates, whereas non-polar solvents (e.g., hexane) are avoided due to poor phase mixing . Catalysts such as crown ethers or quaternary ammonium salts are critical for stabilizing transition states and accelerating reaction rates.
Comparative Data:
Parameter | Value | Source |
---|---|---|
Solvent | Methylene chloride | |
Catalyst | TBAB | |
Reaction Time | 2–3 hours | |
Yield | 89–90% | |
Purity (GC) | >98% |
Synthesis of Di-tert-Butyl this compound
Alkali Metal Carbonate Route
US5151542A discloses a method for preparing DIBOC by reacting alkali metal tert-butyl carbonate (e.g., potassium tert-butoxide) with methanesulfonyl chloride in a non-polar solvent . The reaction mechanism involves the formation of a mixed anhydride intermediate, which undergoes disproportionation to yield DIBOC.
Critical Parameters:
-
Solvent: Hexane or cyclohexane is preferred to avoid side reactions with polar solvents.
-
Stoichiometry: Methanesulfonyl chloride is used at 0.45–0.55 moles per mole of alkali metal carbonate to maximize yield .
-
Temperature: Maintained between -40°C and +40°C to balance reaction rate and stability .
Example Protocol:
In a nitrogen-purged reactor, potassium tert-butoxide (1 mole) is suspended in hexane, and carbon dioxide is bubbled through the mixture to form potassium tert-butyl carbonate. Methanesulfonyl chloride (0.5 moles) is added dropwise at 0–10°C, followed by stirring for 2.5 hours. The organic layer is washed with dilute sulfuric acid and sodium bicarbonate, yielding DIBOC with 89% purity .
Role of Catalysts
Pyridine derivatives, such as N-n-octylpyridinium chloride, enhance reaction efficiency by stabilizing the leaving group (methanesulfonate). Catalytic amounts (0.05 moles) reduce reaction time from 3 hours to 1 hour while increasing purity to 99.3% .
Performance Comparison:
Catalyst | Yield (%) | Purity (%) | Reaction Time (h) |
---|---|---|---|
Pyridine | 89 | 98.6 | 2.5 |
N-n-Octylpyridinium Chloride | 90 | 99.3 | 1.0 |
None | <70 | <95 | >5 |
Mechanistic Insights
Nucleophilic Acyl Substitution
Both DMDC and DIBOC syntheses rely on nucleophilic attack by carbonate ions on electrophilic carbonyl carbons. In DMDC synthesis, methyl chloroformate acts as the acylating agent, while in DIBOC, methanesulfonyl chloride generates a reactive intermediate.
Key Transition States:
-
DMDC: Tetrahedral intermediate formed by attack of CO₃²⁻ on methyl chloroformate .
-
DIBOC: Mixed anhydride (tert-butyl carbonate methanesulfonate) that disproportionates into DIBOC .
Side Reactions and Mitigation
-
Hydrolysis: Unreacted chloroformate or sulfonyl chloride can hydrolyze to carboxylic acids, necessitating anhydrous conditions .
-
Thermal Decomposition: DIBOC degrades above 40°C, requiring strict temperature control .
Industrial-Scale Considerations
Continuous Flow Synthesis
Recent patents (CN107188805A, CN108191659A) describe continuous DMDC production using tubular reactors, which improve heat transfer and reduce batch variability . Key advantages include:
-
Throughput: 500–1,000 kg/day.
-
Purity: >99% by GC.
Emerging Methods
Phase-Transfer Catalysis
CN108794335A introduces a phase-transfer method for di-tert-butyl this compound using tetrabutylammonium iodide, achieving 92% yield in 2 hours . This approach eliminates the need for cryogenic conditions.
Enzymatic Carbonylation
Preliminary studies suggest lipases can catalyze this compound formation under mild conditions, though yields remain low (<50%) .
Properties
CAS No. |
214335-04-3 |
---|---|
Molecular Formula |
C2O5-2 |
Molecular Weight |
104.02 g/mol |
IUPAC Name |
carboxylato carbonate |
InChI |
InChI=1S/C2H2O5/c3-1(4)7-2(5)6/h(H,3,4)(H,5,6)/p-2 |
InChI Key |
ZFTFAPZRGNKQPU-UHFFFAOYSA-L |
SMILES |
C(=O)([O-])OC(=O)[O-] |
Canonical SMILES |
C(=O)([O-])OC(=O)[O-] |
Synonyms |
pyrocarbonate pyrocarbonic acid pyrocarbonic acid, potassium salt |
Origin of Product |
United States |
Disclaimer and Information on In-Vitro Research Products
Please be aware that all articles and product information presented on BenchChem are intended solely for informational purposes. The products available for purchase on BenchChem are specifically designed for in-vitro studies, which are conducted outside of living organisms. In-vitro studies, derived from the Latin term "in glass," involve experiments performed in controlled laboratory settings using cells or tissues. It is important to note that these products are not categorized as medicines or drugs, and they have not received approval from the FDA for the prevention, treatment, or cure of any medical condition, ailment, or disease. We must emphasize that any form of bodily introduction of these products into humans or animals is strictly prohibited by law. It is essential to adhere to these guidelines to ensure compliance with legal and ethical standards in research and experimentation.