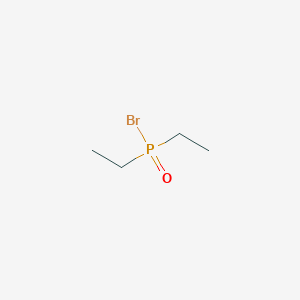
Diethylphosphinic bromide
- Click on QUICK INQUIRY to receive a quote from our team of experts.
- With the quality product at a COMPETITIVE price, you can focus more on your research.
Overview
Description
Diethylphosphinic bromide is an organophosphorus compound characterized by the presence of a phosphorus atom bonded to two ethyl groups and a bromine atom. This compound is of significant interest due to its applications in various chemical reactions and industrial processes.
Preparation Methods
Synthetic Routes and Reaction Conditions: Diethylphosphinic bromide can be synthesized through the reaction of diethylphosphinic acid with bromine. The reaction typically occurs under controlled conditions to ensure the desired product is obtained. The general reaction is as follows:
(C2H5)2P(O)H+Br2→(C2H5)2P(O)Br+HBr
Industrial Production Methods: In industrial settings, the production of this compound involves large-scale reactions with stringent control over temperature and pressure to maximize yield and purity. The use of high-purity reagents and advanced purification techniques is essential to obtain a high-quality product.
Chemical Reactions Analysis
Types of Reactions: Diethylphosphinic bromide undergoes various chemical reactions, including:
Substitution Reactions: It can participate in nucleophilic substitution reactions where the bromine atom is replaced by other nucleophiles.
Oxidation Reactions: The compound can be oxidized to form diethylphosphinic acid or other oxidized derivatives.
Reduction Reactions: Reduction can lead to the formation of diethylphosphine.
Common Reagents and Conditions:
Nucleophiles: Common nucleophiles include hydroxide ions, amines, and alkoxides.
Oxidizing Agents: Hydrogen peroxide and other peroxides are commonly used.
Reducing Agents: Lithium aluminum hydride and other hydride donors are effective.
Major Products:
Diethylphosphinic Acid: Formed through oxidation.
Diethylphosphine: Formed through reduction.
Substituted Phosphinic Compounds: Formed through nucleophilic substitution.
Scientific Research Applications
Diethylphosphinic bromide has diverse applications in scientific research:
Chemistry: Used as a reagent in the synthesis of various organophosphorus compounds.
Biology: Investigated for its potential role in biochemical pathways involving phosphorus-containing compounds.
Medicine: Explored for its potential use in drug development and as a precursor for pharmaceuticals.
Industry: Utilized in the production of flame retardants, plasticizers, and other industrial chemicals.
Mechanism of Action
The mechanism of action of diethylphosphinic bromide involves its reactivity with nucleophiles and electrophiles. The bromine atom acts as a leaving group, facilitating substitution reactions. The phosphorus atom can form bonds with various nucleophiles, leading to the formation of new compounds. The compound’s reactivity is influenced by the electronic and steric effects of the ethyl groups attached to the phosphorus atom.
Comparison with Similar Compounds
Diethylphosphinic Acid: Similar in structure but lacks the bromine atom.
Diethylphosphine: A reduced form of diethylphosphinic bromide.
Triethylphosphine: Contains an additional ethyl group compared to this compound.
Uniqueness: this compound is unique due to the presence of the bromine atom, which imparts distinct reactivity and chemical properties. This makes it a valuable reagent in various chemical transformations that are not possible with its analogs.
Biological Activity
Diethylphosphinic bromide (DEPBr) is an organophosphorus compound that has garnered attention for its potential biological activities, particularly in the fields of toxicology and pharmacology. This article explores the biological activity of DEPBr, including its mechanisms of action, toxicological profiles, and potential therapeutic applications.
This compound has the molecular formula C4H10BrO2P and a molecular weight of approximately 201.00 g/mol. It is characterized by the presence of a phosphorus atom bonded to two ethyl groups and a bromine atom, which contributes to its reactivity and biological interactions.
Mechanisms of Biological Activity
-
Enzyme Inhibition :
- DEPBr has been shown to inhibit various enzymes, particularly acetylcholinesterase (AChE), which is critical in the regulation of neurotransmission. AChE inhibition can lead to an accumulation of acetylcholine at synapses, resulting in prolonged stimulation of cholinergic receptors.
- Studies indicate that DEPBr's interaction with AChE may follow similar pathways as other organophosphorus compounds, leading to neurotoxic effects characterized by symptoms such as muscle twitching, respiratory distress, and convulsions.
-
Cellular Toxicity :
- Research has demonstrated that DEPBr exhibits cytotoxic effects on various cell lines. For instance, it has been reported to induce apoptosis in human neuroblastoma cells through oxidative stress mechanisms.
- The compound's ability to generate reactive oxygen species (ROS) contributes significantly to its cytotoxic profile, leading to cellular damage and death.
Toxicological Profiles
The toxicological effects of DEPBr have been investigated in several studies:
-
Acute Toxicity :
- Animal studies have indicated that acute exposure to DEPBr can result in significant mortality rates due to respiratory failure and central nervous system depression.
- The median lethal dose (LD50) for DEPBr has been estimated to be in the range of 50-100 mg/kg in rodents, indicating high toxicity.
-
Chronic Effects :
- Chronic exposure studies suggest that prolonged contact with DEPBr may lead to neurodegenerative changes, particularly affecting cholinergic neurons.
- Additionally, reproductive toxicity has been noted, with potential impacts on fertility and fetal development in animal models.
Case Studies
Several case studies highlight the real-world implications of DEPBr exposure:
-
Occupational Exposure :
- A case study involving workers in pesticide manufacturing revealed acute symptoms consistent with organophosphate poisoning after accidental exposure to a mixture containing DEPBr. Symptoms included headaches, dizziness, and gastrointestinal disturbances.
-
Environmental Impact :
- Environmental assessments have detected residues of DEPBr in agricultural runoff, raising concerns about its ecological effects on non-target species and potential bioaccumulation in food chains.
Research Findings
Recent research has focused on the synthesis and evaluation of derivatives of DEPBr for potential therapeutic applications:
-
Synthesis of Analogues :
- Novel derivatives have been synthesized to enhance selectivity towards specific biological targets while reducing toxicity. For example, modifications at the ethyl groups have shown promise in improving enzyme inhibitory profiles without compromising safety.
-
Therapeutic Potential :
- Some studies suggest that modified versions of DEPBr could serve as leads for developing new treatments for neurodegenerative diseases by targeting AChE with reduced side effects compared to traditional organophosphates.
Data Summary
Property | Value/Description |
---|---|
Molecular Formula | C4H10BrO2P |
Molecular Weight | 201.00 g/mol |
LD50 (Rodent) | 50-100 mg/kg |
Mechanism of Action | AChE inhibition, oxidative stress |
Acute Symptoms | Respiratory failure, CNS depression |
Chronic Effects | Neurodegeneration, reproductive toxicity |
Properties
CAS No. |
603932-63-4 |
---|---|
Molecular Formula |
C4H10BrOP |
Molecular Weight |
185.00 g/mol |
IUPAC Name |
1-[bromo(ethyl)phosphoryl]ethane |
InChI |
InChI=1S/C4H10BrOP/c1-3-7(5,6)4-2/h3-4H2,1-2H3 |
InChI Key |
JVQNKZPGPIESSJ-UHFFFAOYSA-N |
Canonical SMILES |
CCP(=O)(CC)Br |
Origin of Product |
United States |
Disclaimer and Information on In-Vitro Research Products
Please be aware that all articles and product information presented on BenchChem are intended solely for informational purposes. The products available for purchase on BenchChem are specifically designed for in-vitro studies, which are conducted outside of living organisms. In-vitro studies, derived from the Latin term "in glass," involve experiments performed in controlled laboratory settings using cells or tissues. It is important to note that these products are not categorized as medicines or drugs, and they have not received approval from the FDA for the prevention, treatment, or cure of any medical condition, ailment, or disease. We must emphasize that any form of bodily introduction of these products into humans or animals is strictly prohibited by law. It is essential to adhere to these guidelines to ensure compliance with legal and ethical standards in research and experimentation.