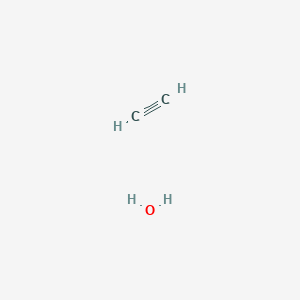
Acetylene-water
- Click on QUICK INQUIRY to receive a quote from our team of experts.
- With the quality product at a COMPETITIVE price, you can focus more on your research.
Overview
Description
Acetylene-water, also known as ethyne-water, is a chemical compound formed by the interaction of acetylene (ethyne) and water. Acetylene is a hydrocarbon with the formula C₂H₂, characterized by a carbon-carbon triple bond. It is a colorless, flammable gas widely used in welding and as a chemical building block . When acetylene reacts with water, it forms acetaldehyde, a key intermediate in various chemical processes .
Synthetic Routes and Reaction Conditions:
-
Laboratory Preparation: Acetylene can be prepared in the laboratory by the action of water on calcium carbide. The reaction is as follows: [ \text{CaC}_2 + 2\text{H}_2\text{O} \rightarrow \text{Ca(OH)}_2 + \text{C}_2\text{H}_2 ] This method involves adding water to calcium carbide in a controlled manner to produce acetylene gas .
-
Industrial Production Methods: Industrially, acetylene is produced by the partial combustion of methane or by passing a hydrocarbon through an electric arc. Another common method is the hydrolysis of calcium carbide .
Types of Reactions:
-
Combustion: Acetylene undergoes combustion in the presence of oxygen to form carbon dioxide and water: [ 2\text{C}_2\text{H}_2 + 5\text{O}_2 \rightarrow 4\text{CO}_2 + 2\text{H}_2\text{O} ] This reaction releases a significant amount of heat and is used in oxyacetylene welding .
-
Addition Reactions: Acetylene can undergo addition reactions with halogens, hydrogen halides, and other reagents to form various products. For example, the addition of hydrogen bromide to acetylene forms bromoethene .
Common Reagents and Conditions:
Catalysts: Mercury(II) sulfate for hydration reactions.
Oxidizing Agents: Oxygen for combustion reactions.
Halogens and Hydrogen Halides: For addition reactions.
Major Products:
Acetaldehyde: From hydration.
Carbon Dioxide and Water: From combustion.
Halogenated Compounds: From addition reactions.
Scientific Research Applications
Acetylene-water and its derivatives have numerous applications in scientific research:
Biology: Acetylene derivatives are used in the synthesis of biologically active molecules and pharmaceuticals.
Medicine: Acetylene compounds are involved in the development of drugs and therapeutic agents.
Industry: Acetylene is used in the production of synthetic rubber, plastics, and other industrial chemicals.
Mechanism of Action
The mechanism of action of acetylene-water involves the hydration of acetylene to form acetaldehyde. This reaction is catalyzed by mercury(II) sulfate, where the acetylene molecule coordinates to the catalyst, and a water molecule performs a nucleophilic attack on the acetylene, resulting in the formation of a vinyl anion intermediate. This intermediate is then protonated to form acetaldehyde .
Comparison with Similar Compounds
Ethylene (C₂H₄): Unlike acetylene, ethylene has a carbon-carbon double bond and undergoes different types of reactions, such as polymerization to form polyethylene.
Propylene (C₃H₆): Propylene has a similar structure to ethylene but with an additional methyl group, leading to different reactivity and applications.
Uniqueness of Acetylene-Water:
Triple Bond Reactivity: The carbon-carbon triple bond in acetylene makes it highly reactive and versatile in chemical synthesis compared to compounds with double bonds.
High Energy Release: The combustion of acetylene releases a significant amount of heat, making it useful in welding and cutting applications.
This compound is a compound with diverse applications and unique chemical properties, making it a valuable subject of study in various scientific fields.
Properties
CAS No. |
164078-76-6 |
---|---|
Molecular Formula |
C2H4O |
Molecular Weight |
44.05 g/mol |
IUPAC Name |
acetylene;hydrate |
InChI |
InChI=1S/C2H2.H2O/c1-2;/h1-2H;1H2 |
InChI Key |
HMYNUKPKYAKNHH-UHFFFAOYSA-N |
Canonical SMILES |
C#C.O |
Origin of Product |
United States |
Disclaimer and Information on In-Vitro Research Products
Please be aware that all articles and product information presented on BenchChem are intended solely for informational purposes. The products available for purchase on BenchChem are specifically designed for in-vitro studies, which are conducted outside of living organisms. In-vitro studies, derived from the Latin term "in glass," involve experiments performed in controlled laboratory settings using cells or tissues. It is important to note that these products are not categorized as medicines or drugs, and they have not received approval from the FDA for the prevention, treatment, or cure of any medical condition, ailment, or disease. We must emphasize that any form of bodily introduction of these products into humans or animals is strictly prohibited by law. It is essential to adhere to these guidelines to ensure compliance with legal and ethical standards in research and experimentation.