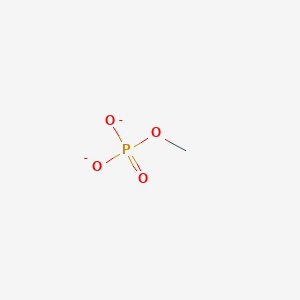
Monomethyl phosphate
- Click on QUICK INQUIRY to receive a quote from our team of experts.
- With the quality product at a COMPETITIVE price, you can focus more on your research.
Overview
Description
Methyl phosphate(2-) is dianion of methyl phosphate arising from deprotonation of both OH groups of the phosphate. It has a role as a phosphoantigen and an epitope. It is a conjugate base of a methyl dihydrogen phosphate.
Scientific Research Applications
Phosphoester Hydrolysis in Biological Systems
Monomethyl phosphate is pivotal in understanding the hydrolysis of phosphate monoesters, a key biological reaction. Investigations using hybrid quantum mechanical and molecular mechanical simulations revealed detailed free energy landscapes of this process. This is particularly significant for understanding biological mechanisms involving phosphate groups (Li et al., 2012).
Biomembrane Adhesion
This compound derivatives have been used in creating a universal biomembrane adhesive. This adhesive exhibits rapid attachment to mammalian cell membranes and is valuable in tissue engineering and drug delivery applications (Yu et al., 2013).
Phosphorus Source for Plant Growth
Various phosphates, including this compound, have been evaluated as phosphorus sources for plant growth. These studies are crucial for understanding the bioavailability of different phosphorus sources in agriculture (Johnston & Richards, 2003).
Plasma Polymer Coatings
Research on plasma polymer coatings containing phosphorus, like this compound, has shown their potential in bio-interfacial applications. This is relevant in the development of biomaterials and medical implants (Siow et al., 2014).
Protein Modification and Artificial Blood
This compound esters have been studied for their potential in protein modification and artificial blood applications. This research is significant for biomedical applications, including transfusion medicine (Kluger, 1994).
Polymer Synthesis and Biomedical Applications
Studies have explored the ring-opening polymerization of cyclic ethylene phosphate monomers, including this compound, for biomedical applications. This polymerization process is key in developing new materials for medical use (Nifant’ev et al., 2018).
Addressing Phosphate Crisis
Research has highlighted the critical role of phosphates, including this compound, in global agriculture and the impending phosphate crisis. This is vital for sustainable agriculture and environmental conservation (Abelson, 1999).
Biological Interactions Study Model
This compound's interaction with biogenic amines offers a model for studying biological interactions, relevant in pharmacology and neuroscience (Katz et al., 1977).
Environmental Applications
Research on this compound adsorption to soil minerals like goethite informs us about its role in the global phosphorus cycle, which is crucial for environmental science and soil chemistry (Persson et al., 2012).
Effects on Human Erythrocytes
Studies have explored the effects of phosphates on the oxygen equilibrium of human erythrocytes, contributing to our understanding of blood chemistry and physiology (Chanutin & Curnish, 1967).
Properties
Molecular Formula |
CH3O4P-2 |
---|---|
Molecular Weight |
110.01 g/mol |
IUPAC Name |
methyl phosphate |
InChI |
InChI=1S/CH5O4P/c1-5-6(2,3)4/h1H3,(H2,2,3,4)/p-2 |
InChI Key |
CAAULPUQFIIOTL-UHFFFAOYSA-L |
SMILES |
COP(=O)([O-])[O-] |
Canonical SMILES |
COP(=O)([O-])[O-] |
synonyms |
methyl phosphate disodium salt, hexahydrate methylphosphate methylphosphate diammonium salt methylphosphate diammonium salt, dihydrate methylphosphate dipotassium salt methylphosphate monoammonium salt methylphosphate monopotassium salt methylphosphate monosodium salt methylphosphate vanadium salt methylphosphate, phosporic-(17)O-(18)O acid (16)O-methyl ester, disodium salt, (R)-isomer monomethyl phosphate |
Origin of Product |
United States |
Retrosynthesis Analysis
AI-Powered Synthesis Planning: Our tool employs the Template_relevance Pistachio, Template_relevance Bkms_metabolic, Template_relevance Pistachio_ringbreaker, Template_relevance Reaxys, Template_relevance Reaxys_biocatalysis model, leveraging a vast database of chemical reactions to predict feasible synthetic routes.
One-Step Synthesis Focus: Specifically designed for one-step synthesis, it provides concise and direct routes for your target compounds, streamlining the synthesis process.
Accurate Predictions: Utilizing the extensive PISTACHIO, BKMS_METABOLIC, PISTACHIO_RINGBREAKER, REAXYS, REAXYS_BIOCATALYSIS database, our tool offers high-accuracy predictions, reflecting the latest in chemical research and data.
Strategy Settings
Precursor scoring | Relevance Heuristic |
---|---|
Min. plausibility | 0.01 |
Model | Template_relevance |
Template Set | Pistachio/Bkms_metabolic/Pistachio_ringbreaker/Reaxys/Reaxys_biocatalysis |
Top-N result to add to graph | 6 |
Feasible Synthetic Routes
Disclaimer and Information on In-Vitro Research Products
Please be aware that all articles and product information presented on BenchChem are intended solely for informational purposes. The products available for purchase on BenchChem are specifically designed for in-vitro studies, which are conducted outside of living organisms. In-vitro studies, derived from the Latin term "in glass," involve experiments performed in controlled laboratory settings using cells or tissues. It is important to note that these products are not categorized as medicines or drugs, and they have not received approval from the FDA for the prevention, treatment, or cure of any medical condition, ailment, or disease. We must emphasize that any form of bodily introduction of these products into humans or animals is strictly prohibited by law. It is essential to adhere to these guidelines to ensure compliance with legal and ethical standards in research and experimentation.