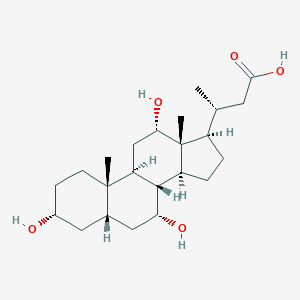
Norcholic acid
Overview
Description
Norcholic acid is a bile acid, a class of molecules derived from cholesterol in the liver. Bile acids play a crucial role in the digestion and absorption of fats and fat-soluble vitamins in the small intestine. This compound is not naturally occurring but is found in individuals exposed to this compound or its derivatives . It has been identified as a novel biomarker for early kidney injury in patients with metabolic dysfunction-associated fatty liver disease .
Mechanism of Action
Target of Action
Norcholic acid (NorCA) primarily targets the Farnesoid X receptor (FXR) . FXR is a nuclear receptor that plays a crucial role in regulating bile acid levels in the liver and intestines . It is also involved in the regulation of lipid and glucose metabolism .
Mode of Action
NorCA interacts with FXR, leading to its negative regulation . This interaction results in the promotion of hepatocellular carcinoma (HCC) cell proliferation, migration, and invasion . Additionally, NorCA can increase the level of PD-L1 on the surfaces of HCC cells and their exosomes .
Biochemical Pathways
The interaction of NorCA with FXR affects various biochemical pathways. The dysregulation of FXR signaling by NorCA promotes the progression of HCC and immune invasion . NorCA-induced exosomes dramatically dampen the function of CD4+ T cells, thereby inducing an immunosuppressive microenvironment .
Pharmacokinetics
It is known that bile acids, including norca, are metabolites generated in the liver and synthesized from cholesterol via both the nonclassical and classical pathways .
Result of Action
The result of NorCA’s action is the promotion of HCC progression and immune invasion through the inhibition of FXR signaling . NorCA can increase the level of PD-L1 on the surfaces of HCC cells and their exosomes, leading to an immunosuppressive microenvironment . A negative correlation between PD-L1 and FXR expression in human HCC specimens has been identified, and HCC patients with low FXR and high PD-L1 expression exhibit a rather dismal survival outcome .
Action Environment
The action of NorCA can be influenced by various environmental factors.
Biochemical Analysis
Biochemical Properties
Norcholic acid plays a significant role in biochemical reactions, particularly in the metabolism of bile acids. It interacts with various enzymes, proteins, and other biomolecules. One of the key enzymes it interacts with is sterol 27-hydroxylase (CYP27A1), which is involved in the classical pathway of bile acid synthesis. This compound is efficiently absorbed from the intestine and quickly excreted into the bile but not into the urine . This interaction is crucial for maintaining bile acid homeostasis and regulating metabolic processes.
Cellular Effects
This compound has been shown to influence various cellular processes, particularly in hepatocellular carcinoma (HCC) cells. It promotes HCC cell proliferation, migration, and invasion by negatively regulating the Farnesoid X receptor (FXR). Additionally, this compound increases the level of PD-L1 on the surfaces of HCC cells and their exosomes, which dampens the function of CD4+ T cells and induces an immunosuppressive microenvironment . This indicates that this compound plays a role in tumor progression and immune escape.
Molecular Mechanism
At the molecular level, this compound exerts its effects by binding to and inhibiting the Farnesoid X receptor (FXR). This inhibition leads to the upregulation of PD-L1 on HCC cells, which in turn promotes immune escape and tumor progression. The interaction between this compound and FXR is a key mechanism through which it influences gene expression and cellular signaling pathways . Additionally, this compound-induced exosomes significantly dampen the function of CD4+ T cells, further contributing to an immunosuppressive environment.
Temporal Effects in Laboratory Settings
In laboratory settings, the effects of this compound have been observed to change over time. Studies have shown that this compound is efficiently absorbed from the intestine and quickly excreted into the bile within 24 hours . This rapid excretion suggests that this compound has a short half-life and may require continuous administration to maintain its effects. Long-term studies are needed to fully understand the stability, degradation, and long-term effects of this compound on cellular function.
Dosage Effects in Animal Models
The effects of this compound vary with different dosages in animal models. In a study involving male Wistar rats, an oral dosage of 6.4 g/L of this compound resulted in an average recovery of 40% of the administered radioactivity from the feces within the first 24 hours This indicates that this compound is efficiently absorbed and excreted
Metabolic Pathways
This compound is involved in the metabolic pathways of bile acids. It is synthesized from cholesterol via both the nonclassical and classical pathways, which are under the control of specific enzymes such as sterol 27-hydroxylase (CYP27A1) . The dysmetabolism of bile acids, including this compound, can promote the development of hepatocellular carcinoma associated with obesity or fatty liver . Understanding the metabolic pathways of this compound is crucial for developing therapeutic strategies targeting bile acid metabolism.
Transport and Distribution
This compound is transported and distributed within cells and tissues primarily through the bile. It is efficiently absorbed from the intestine and quickly excreted into the bile but not into the urine . This suggests that this compound is primarily localized in the liver and bile ducts, where it exerts its effects on bile acid metabolism and cellular function.
Subcellular Localization
The subcellular localization of this compound is primarily within the liver cells, particularly in the bile ducts. It interacts with the Farnesoid X receptor (FXR) within the cells, leading to changes in gene expression and cellular signaling pathways
Preparation Methods
Norcholic acid can be synthesized through various methods. One approach involves the degradation of the side chain of natural bile acids, removing a methyl group . Another method includes the in vitro incubation of 23-nordeoxycholic acid with enzyme-enriched liver subcellular fractions of mouse, rat, or human . The metabolic pathways involved in the synthesis include hydroxylation, oxidation, epimerization, sulfation, and glucuronidation .
Chemical Reactions Analysis
Norcholic acid undergoes several types of chemical reactions:
Oxidation: This reaction involves the addition of oxygen or the removal of hydrogen. Common reagents include oxidizing agents like potassium permanganate or chromium trioxide.
Reduction: This reaction involves the addition of hydrogen or the removal of oxygen. Common reagents include reducing agents like lithium aluminum hydride or sodium borohydride.
Substitution: This reaction involves the replacement of one atom or group of atoms with another. Common reagents include halogens or nucleophiles.
Hydroxylation: This reaction involves the addition of a hydroxyl group. Common reagents include hydroxylating agents like hydrogen peroxide or osmium tetroxide.
The major products formed from these reactions include various hydroxylated, oxidized, and reduced derivatives of this compound .
Scientific Research Applications
Norcholic acid has several scientific research applications:
Chemistry: It is used as a model compound to study the metabolism and transformation of bile acids.
Industry: It is used in the development of diagnostic tools and therapeutic agents for liver and kidney diseases.
Comparison with Similar Compounds
Norcholic acid is similar to other bile acids such as cholic acid, tauro-deoxycholic acid, and tauro-lithocholic acid . it is unique in its ability to serve as a biomarker for early kidney injury and its role in promoting tumor progression and immune escape . The structural differences between this compound and other bile acids include the removal of a methyl group and the presence of additional hydroxyl groups .
References
Properties
IUPAC Name |
(3R)-3-[(3R,5S,7R,8R,9S,10S,12S,13R,14S,17R)-3,7,12-trihydroxy-10,13-dimethyl-2,3,4,5,6,7,8,9,11,12,14,15,16,17-tetradecahydro-1H-cyclopenta[a]phenanthren-17-yl]butanoic acid | |
---|---|---|
Source | PubChem | |
URL | https://pubchem.ncbi.nlm.nih.gov | |
Description | Data deposited in or computed by PubChem | |
InChI |
InChI=1S/C23H38O5/c1-12(8-20(27)28)15-4-5-16-21-17(11-19(26)23(15,16)3)22(2)7-6-14(24)9-13(22)10-18(21)25/h12-19,21,24-26H,4-11H2,1-3H3,(H,27,28)/t12-,13+,14-,15-,16+,17+,18-,19+,21+,22+,23-/m1/s1 | |
Source | PubChem | |
URL | https://pubchem.ncbi.nlm.nih.gov | |
Description | Data deposited in or computed by PubChem | |
InChI Key |
SHUYNJFEXPRUGR-RTCCEZQESA-N | |
Source | PubChem | |
URL | https://pubchem.ncbi.nlm.nih.gov | |
Description | Data deposited in or computed by PubChem | |
Canonical SMILES |
CC(CC(=O)O)C1CCC2C1(C(CC3C2C(CC4C3(CCC(C4)O)C)O)O)C | |
Source | PubChem | |
URL | https://pubchem.ncbi.nlm.nih.gov | |
Description | Data deposited in or computed by PubChem | |
Isomeric SMILES |
C[C@H](CC(=O)O)[C@H]1CC[C@@H]2[C@@]1([C@H](C[C@H]3[C@H]2[C@@H](C[C@H]4[C@@]3(CC[C@H](C4)O)C)O)O)C | |
Source | PubChem | |
URL | https://pubchem.ncbi.nlm.nih.gov | |
Description | Data deposited in or computed by PubChem | |
Molecular Formula |
C23H38O5 | |
Source | PubChem | |
URL | https://pubchem.ncbi.nlm.nih.gov | |
Description | Data deposited in or computed by PubChem | |
Molecular Weight |
394.5 g/mol | |
Source | PubChem | |
URL | https://pubchem.ncbi.nlm.nih.gov | |
Description | Data deposited in or computed by PubChem | |
CAS No. |
60696-62-0 | |
Record name | Norcholic acid | |
Source | ChemIDplus | |
URL | https://pubchem.ncbi.nlm.nih.gov/substance/?source=chemidplus&sourceid=0060696620 | |
Description | ChemIDplus is a free, web search system that provides access to the structure and nomenclature authority files used for the identification of chemical substances cited in National Library of Medicine (NLM) databases, including the TOXNET system. | |
Retrosynthesis Analysis
AI-Powered Synthesis Planning: Our tool employs the Template_relevance Pistachio, Template_relevance Bkms_metabolic, Template_relevance Pistachio_ringbreaker, Template_relevance Reaxys, Template_relevance Reaxys_biocatalysis model, leveraging a vast database of chemical reactions to predict feasible synthetic routes.
One-Step Synthesis Focus: Specifically designed for one-step synthesis, it provides concise and direct routes for your target compounds, streamlining the synthesis process.
Accurate Predictions: Utilizing the extensive PISTACHIO, BKMS_METABOLIC, PISTACHIO_RINGBREAKER, REAXYS, REAXYS_BIOCATALYSIS database, our tool offers high-accuracy predictions, reflecting the latest in chemical research and data.
Strategy Settings
Precursor scoring | Relevance Heuristic |
---|---|
Min. plausibility | 0.01 |
Model | Template_relevance |
Template Set | Pistachio/Bkms_metabolic/Pistachio_ringbreaker/Reaxys/Reaxys_biocatalysis |
Top-N result to add to graph | 6 |
Feasible Synthetic Routes
Disclaimer and Information on In-Vitro Research Products
Please be aware that all articles and product information presented on BenchChem are intended solely for informational purposes. The products available for purchase on BenchChem are specifically designed for in-vitro studies, which are conducted outside of living organisms. In-vitro studies, derived from the Latin term "in glass," involve experiments performed in controlled laboratory settings using cells or tissues. It is important to note that these products are not categorized as medicines or drugs, and they have not received approval from the FDA for the prevention, treatment, or cure of any medical condition, ailment, or disease. We must emphasize that any form of bodily introduction of these products into humans or animals is strictly prohibited by law. It is essential to adhere to these guidelines to ensure compliance with legal and ethical standards in research and experimentation.