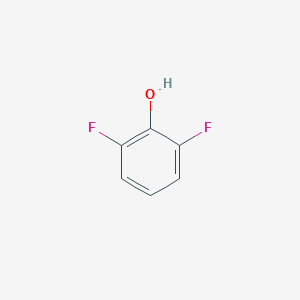
2,6-Difluorophenol
Overview
Description
Mechanism of Action
Target of Action
It’s known that this compound is used as a building block in various chemical reactions .
Mode of Action
2,6-Difluorophenol undergoes oxidative polymerization in the presence of the Fe-N,N′-bis(salicylidene)ethylenediamine (salen) complex (catalyst) and hydrogen peroxide (oxidizing agent) to give poly(2,6-difluoro-1,4-phenylene oxide) . This process involves the transformation of the this compound molecule into a polymer, altering its chemical structure and properties.
Biochemical Pathways
The compound is known to undergo a regioselective difunctionalization process with aryl sulfoxides and nucleophiles . This process involves a Pummerer-based [3,3] sigmatropic dearomatization to generate 2,4-cyclohexadienone, followed by a Michael addition of a nucleophile, and finally, liberation of HF for rearomatization .
Pharmacokinetics
It’s soluble in ethanol , which could potentially influence its bioavailability and distribution in an organism.
Result of Action
The result of the action of this compound is the production of poly(2,6-difluoro-1,4-phenylene oxide) through oxidative polymerization . This polymer could have various applications depending on its physical and chemical properties.
Action Environment
The action of this compound can be influenced by various environmental factors. For instance, the oxidative polymerization process requires the presence of a catalyst and an oxidizing agent . Additionally, the compound should be stored at a temperature of 2-8°C and protected from light , indicating that temperature and light exposure could affect its stability and efficacy.
Biochemical Analysis
Biochemical Properties
It is known that 2,6-Difluorophenol can undergo oxidative polymerization in the presence of the Fe-N,N′-bis(salicylidene)ethylenediamine (salen) complex (catalyst) and hydrogen peroxide (oxidizing agent) to give poly(2,6-difluoro-1,4-phenylene oxide)
Cellular Effects
It is known that the compound can cause irritation to the respiratory system
Molecular Mechanism
It is known that the compound can undergo oxidative polymerization
Preparation Methods
Glyceryl monolinoleate is typically obtained through the partial glycerolysis of vegetable oils rich in triglycerides of linoleic acid . The process involves the following steps:
Glycerolysis: Vegetable oil is reacted with glycerol under controlled conditions to produce a mixture of mono-, di-, and triglycerides.
Industrial production methods often include the addition of suitable antioxidants to stabilize the compound and prevent oxidation .
Chemical Reactions Analysis
Glyceryl monolinoleate undergoes various chemical reactions, including:
Hydrolysis: In the presence of water and enzymes, glyceryl monolinoleate can hydrolyze to produce glycerol and linoleic acid.
Esterification: The compound can undergo esterification reactions with other fatty acids to form different esters.
Common reagents used in these reactions include oxygen for oxidation, water and enzymes for hydrolysis, and fatty acids for esterification. The major products formed from these reactions are peroxides, glycerol, linoleic acid, and various esters .
Scientific Research Applications
Glyceryl monolinoleate has a wide range of applications in scientific research, including:
Comparison with Similar Compounds
Glyceryl monolinoleate can be compared with other similar compounds such as glyceryl monooleate, glyceryl monostearate, and glyceryl monocaprylate . While all these compounds are used as solubilizers and bioavailability enhancers, glyceryl monolinoleate is unique due to its high content of linoleic acid, which imparts specific properties such as enhanced lipid metabolism and anti-inflammatory effects .
Similar Compounds
- Glyceryl monooleate
- Glyceryl monostearate
- Glyceryl monocaprylate
Glyceryl monolinoleate stands out for its specific applications in lipid-based drug delivery systems and its ability to enhance the bioavailability of poorly water-soluble drugs .
Properties
IUPAC Name |
2,6-difluorophenol | |
---|---|---|
Source | PubChem | |
URL | https://pubchem.ncbi.nlm.nih.gov | |
Description | Data deposited in or computed by PubChem | |
InChI |
InChI=1S/C6H4F2O/c7-4-2-1-3-5(8)6(4)9/h1-3,9H | |
Source | PubChem | |
URL | https://pubchem.ncbi.nlm.nih.gov | |
Description | Data deposited in or computed by PubChem | |
InChI Key |
CKKOVFGIBXCEIJ-UHFFFAOYSA-N | |
Source | PubChem | |
URL | https://pubchem.ncbi.nlm.nih.gov | |
Description | Data deposited in or computed by PubChem | |
Canonical SMILES |
C1=CC(=C(C(=C1)F)O)F | |
Source | PubChem | |
URL | https://pubchem.ncbi.nlm.nih.gov | |
Description | Data deposited in or computed by PubChem | |
Molecular Formula |
C6H4F2O | |
Source | PubChem | |
URL | https://pubchem.ncbi.nlm.nih.gov | |
Description | Data deposited in or computed by PubChem | |
DSSTOX Substance ID |
DTXSID00182456 | |
Record name | 2,6-Difluorophenol | |
Source | EPA DSSTox | |
URL | https://comptox.epa.gov/dashboard/DTXSID00182456 | |
Description | DSSTox provides a high quality public chemistry resource for supporting improved predictive toxicology. | |
Molecular Weight |
130.09 g/mol | |
Source | PubChem | |
URL | https://pubchem.ncbi.nlm.nih.gov | |
Description | Data deposited in or computed by PubChem | |
CAS No. |
28177-48-2 | |
Record name | 2,6-Difluorophenol | |
Source | CAS Common Chemistry | |
URL | https://commonchemistry.cas.org/detail?cas_rn=28177-48-2 | |
Description | CAS Common Chemistry is an open community resource for accessing chemical information. Nearly 500,000 chemical substances from CAS REGISTRY cover areas of community interest, including common and frequently regulated chemicals, and those relevant to high school and undergraduate chemistry classes. This chemical information, curated by our expert scientists, is provided in alignment with our mission as a division of the American Chemical Society. | |
Explanation | The data from CAS Common Chemistry is provided under a CC-BY-NC 4.0 license, unless otherwise stated. | |
Record name | 2,6-Difluorophenol | |
Source | ChemIDplus | |
URL | https://pubchem.ncbi.nlm.nih.gov/substance/?source=chemidplus&sourceid=0028177482 | |
Description | ChemIDplus is a free, web search system that provides access to the structure and nomenclature authority files used for the identification of chemical substances cited in National Library of Medicine (NLM) databases, including the TOXNET system. | |
Record name | 2,6-Difluorophenol | |
Source | EPA DSSTox | |
URL | https://comptox.epa.gov/dashboard/DTXSID00182456 | |
Description | DSSTox provides a high quality public chemistry resource for supporting improved predictive toxicology. | |
Record name | 2,6-difluorophenol | |
Source | European Chemicals Agency (ECHA) | |
URL | https://echa.europa.eu/substance-information/-/substanceinfo/100.044.426 | |
Description | The European Chemicals Agency (ECHA) is an agency of the European Union which is the driving force among regulatory authorities in implementing the EU's groundbreaking chemicals legislation for the benefit of human health and the environment as well as for innovation and competitiveness. | |
Explanation | Use of the information, documents and data from the ECHA website is subject to the terms and conditions of this Legal Notice, and subject to other binding limitations provided for under applicable law, the information, documents and data made available on the ECHA website may be reproduced, distributed and/or used, totally or in part, for non-commercial purposes provided that ECHA is acknowledged as the source: "Source: European Chemicals Agency, http://echa.europa.eu/". Such acknowledgement must be included in each copy of the material. ECHA permits and encourages organisations and individuals to create links to the ECHA website under the following cumulative conditions: Links can only be made to webpages that provide a link to the Legal Notice page. | |
Retrosynthesis Analysis
AI-Powered Synthesis Planning: Our tool employs the Template_relevance Pistachio, Template_relevance Bkms_metabolic, Template_relevance Pistachio_ringbreaker, Template_relevance Reaxys, Template_relevance Reaxys_biocatalysis model, leveraging a vast database of chemical reactions to predict feasible synthetic routes.
One-Step Synthesis Focus: Specifically designed for one-step synthesis, it provides concise and direct routes for your target compounds, streamlining the synthesis process.
Accurate Predictions: Utilizing the extensive PISTACHIO, BKMS_METABOLIC, PISTACHIO_RINGBREAKER, REAXYS, REAXYS_BIOCATALYSIS database, our tool offers high-accuracy predictions, reflecting the latest in chemical research and data.
Strategy Settings
Precursor scoring | Relevance Heuristic |
---|---|
Min. plausibility | 0.01 |
Model | Template_relevance |
Template Set | Pistachio/Bkms_metabolic/Pistachio_ringbreaker/Reaxys/Reaxys_biocatalysis |
Top-N result to add to graph | 6 |
Feasible Synthetic Routes
ANone: Research suggests that 2,6-difluorophenol acts as a lipophilic bioisostere of carboxylic acid, mimicking its interactions. [, ] In studies with pig brain GABA aminotransferase, this compound derivatives demonstrated poor substrate activity but exhibited inhibitory effects. [] This suggests its potential as a scaffold for developing GABA receptor antagonists with enhanced lipophilicity for improved drug candidates. [, ] Further research explored aminomethyl-2,6-difluorophenols as potential GABAc receptor antagonists. [, ]
ANone: this compound has the molecular formula C6H4F2O and a molecular weight of 130.09 g/mol. [] Spectroscopic studies provide detailed insights into its structure and properties. For instance, gas-phase electron diffraction studies determined its molecular structure, revealing a potential weak intramolecular hydrogen bond between the hydroxyl (OH) and fluorine (F) substituents. [, ] Infrared and near-infrared spectroscopy revealed hydrogen bonding effects and solvent dependencies on the wavenumbers and absorption intensities of OH stretching vibrations. [] These findings contribute to a comprehensive understanding of this compound's behavior in various environments.
ANone: The stability and material compatibility of this compound are crucial for its applications. Studies have explored its use as a monomer in the synthesis of poly(phenylene oxide), a valuable engineering plastic. [, , , ] Oxidative polymerization of this compound, catalyzed by horseradish peroxidase or copper-containing bimetallic complexes, yielded polymers with promising properties, including solubility in organic solvents and water repellency. [, , ] These findings highlight the potential of this compound in developing advanced materials.
ANone: While this compound itself may not exhibit direct catalytic activity, its incorporation into metal complexes can influence catalytic processes. For instance, studies demonstrated that copper(II) complexes with 2,6-difluorophenoxide ligands effectively catalyzed the aerobic oxidative coupling polymerization of this compound. [] The presence of 2,6-difluorophenoxide ligands modulated the reactivity and selectivity of the copper catalyst, affecting the molecular weight and properties of the resulting polymers. [, ]
ANone: Computational chemistry plays a crucial role in investigating the properties and behavior of this compound. Ab initio molecular orbital calculations have been utilized to study the intramolecular hydrogen bonding in this compound and related fluorophenol derivatives. [] These calculations provide valuable insights into the strength and nature of hydrogen bonding interactions, which influence the compound's reactivity and physical properties. [, ] Quantum chemical calculations, combined with experimental techniques like photoelectron spectroscopy, have also been employed to explore the electronic structure and excited state dynamics of this compound. []
ANone: Understanding the SAR of this compound is crucial for designing derivatives with tailored properties. Research on bioisosteric analogues of GABA, incorporating the this compound moiety, highlights the impact of structural modifications on activity and potency. [, , ] For instance, introducing aminomethyl groups at different positions on the this compound ring significantly influenced its interaction with GABA receptors, affecting its substrate or inhibitory properties. [] Similarly, in silico studies exploring this compound and squaric acid motifs as carboxylic acid bioisosteres in GPR40 agonists demonstrated the influence of structural modifications on binding affinity and potential therapeutic applications in diabetes treatment. []
Disclaimer and Information on In-Vitro Research Products
Please be aware that all articles and product information presented on BenchChem are intended solely for informational purposes. The products available for purchase on BenchChem are specifically designed for in-vitro studies, which are conducted outside of living organisms. In-vitro studies, derived from the Latin term "in glass," involve experiments performed in controlled laboratory settings using cells or tissues. It is important to note that these products are not categorized as medicines or drugs, and they have not received approval from the FDA for the prevention, treatment, or cure of any medical condition, ailment, or disease. We must emphasize that any form of bodily introduction of these products into humans or animals is strictly prohibited by law. It is essential to adhere to these guidelines to ensure compliance with legal and ethical standards in research and experimentation.