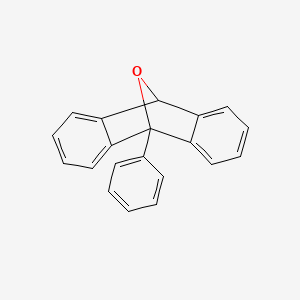
9,10-Epoxyanthracene, 9,10-dihydro-9-phenyl-
- Click on QUICK INQUIRY to receive a quote from our team of experts.
- With the quality product at a COMPETITIVE price, you can focus more on your research.
Overview
Description
9,10-Epoxyanthracene, 9,10-dihydro-9-phenyl- is a chemical compound belonging to the class of anthracenes. Anthracenes are polycyclic aromatic hydrocarbons known for their applications in various fields such as organic electronics, photophysics, and materials science. This compound is characterized by the presence of an epoxide group at the 9,10-positions and a phenyl group at the 9-position, making it a unique derivative of anthracene.
Preparation Methods
Synthetic Routes and Reaction Conditions
The synthesis of 9,10-Epoxyanthracene, 9,10-dihydro-9-phenyl- typically involves the epoxidation of anthracene derivatives. One common method is the reaction of 9,10-dihydro-9-phenylanthracene with a peracid, such as m-chloroperbenzoic acid (m-CPBA), under controlled conditions. The reaction is usually carried out in an organic solvent like dichloromethane at low temperatures to ensure the selective formation of the epoxide group.
Industrial Production Methods
While specific industrial production methods for 9,10-Epoxyanthracene, 9,10-dihydro-9-phenyl- are not widely documented, the general approach involves scaling up the laboratory synthesis methods. This includes optimizing reaction conditions, using high-purity reagents, and employing efficient purification techniques such as recrystallization or chromatography to obtain the desired product in large quantities.
Chemical Reactions Analysis
Types of Reactions
9,10-Epoxyanthracene, 9,10-dihydro-9-phenyl- undergoes various chemical reactions, including:
Oxidation: The epoxide group can be further oxidized to form diols or other oxygenated derivatives.
Reduction: The compound can be reduced to form the corresponding dihydroanthracene derivative.
Substitution: The phenyl group can participate in electrophilic aromatic substitution reactions, leading to the formation of various substituted derivatives.
Common Reagents and Conditions
Oxidation: Reagents like hydrogen peroxide or peracids are commonly used for oxidation reactions.
Reduction: Reducing agents such as lithium aluminum hydride (LiAlH4) or sodium borohydride (NaBH4) are employed.
Substitution: Electrophilic reagents like bromine or nitric acid can be used for substitution reactions.
Major Products
The major products formed from these reactions include diols, reduced anthracene derivatives, and various substituted anthracenes, depending on the specific reaction conditions and reagents used.
Scientific Research Applications
9,10-Epoxyanthracene, 9,10-dihydro-9-phenyl- has several applications in scientific research:
Chemistry: It is used as a precursor for the synthesis of more complex organic molecules and as a model compound for studying epoxide chemistry.
Biology: The compound’s derivatives are investigated for their potential biological activities, including anticancer and antimicrobial properties.
Medicine: Research is ongoing to explore its potential use in drug development and as a probe for studying biological processes.
Industry: It finds applications in the development of organic semiconductors, light-emitting diodes (OLEDs), and other electronic materials.
Mechanism of Action
The mechanism of action of 9,10-Epoxyanthracene, 9,10-dihydro-9-phenyl- involves its interaction with molecular targets through its epoxide and phenyl groups. The epoxide group can form covalent bonds with nucleophilic sites in biological molecules, leading to various biochemical effects. The phenyl group can participate in π-π interactions and other non-covalent interactions, influencing the compound’s overall activity and properties.
Comparison with Similar Compounds
Similar Compounds
9,10-Diphenylanthracene: A similar compound with two phenyl groups at the 9,10-positions, known for its use in OLEDs and as a scintillator material.
9,10-Dimethylanthracene: Another derivative with methyl groups at the 9,10-positions, used in photophysical studies and as a fluorescent probe.
9,10-Dihydroanthracene: A simpler derivative without the phenyl or epoxide groups, used as a starting material for various chemical syntheses.
Uniqueness
9,10-Epoxyanthracene, 9,10-dihydro-9-phenyl- is unique due to the presence of both an epoxide and a phenyl group, which confer distinct chemical reactivity and potential applications. Its ability to undergo a variety of chemical reactions and its potential use in diverse fields make it a valuable compound for scientific research and industrial applications.
Properties
CAS No. |
142230-36-2 |
---|---|
Molecular Formula |
C20H14O |
Molecular Weight |
270.3 g/mol |
IUPAC Name |
1-phenyl-15-oxatetracyclo[6.6.1.02,7.09,14]pentadeca-2,4,6,9,11,13-hexaene |
InChI |
InChI=1S/C20H14O/c1-2-8-14(9-3-1)20-17-12-6-4-10-15(17)19(21-20)16-11-5-7-13-18(16)20/h1-13,19H |
InChI Key |
GNJGRRUTMQGBFZ-UHFFFAOYSA-N |
Canonical SMILES |
C1=CC=C(C=C1)C23C4=CC=CC=C4C(O2)C5=CC=CC=C35 |
Origin of Product |
United States |
Disclaimer and Information on In-Vitro Research Products
Please be aware that all articles and product information presented on BenchChem are intended solely for informational purposes. The products available for purchase on BenchChem are specifically designed for in-vitro studies, which are conducted outside of living organisms. In-vitro studies, derived from the Latin term "in glass," involve experiments performed in controlled laboratory settings using cells or tissues. It is important to note that these products are not categorized as medicines or drugs, and they have not received approval from the FDA for the prevention, treatment, or cure of any medical condition, ailment, or disease. We must emphasize that any form of bodily introduction of these products into humans or animals is strictly prohibited by law. It is essential to adhere to these guidelines to ensure compliance with legal and ethical standards in research and experimentation.