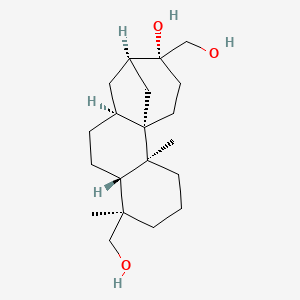
3-Deoxyaphidicolin
Overview
Description
3-Deoxyaphidicolin is a diterpenoid compound derived from aphidicolin, a naturally occurring tetracyclic diterpenoid. It is known for its unique structure and significant biological activities, particularly its role as an inhibitor of DNA polymerase α. This compound is produced by certain fungi, including Cephalosporium aphidicola and Phoma betae .
Preparation Methods
Synthetic Routes and Reaction Conditions: The synthesis of 3-Deoxyaphidicolin involves the heterologous expression of biosynthetic genes in fungal hosts. The genes responsible for the biosynthesis of aphidicolin, including geranylgeranyl diphosphate synthase, terpene synthase, and monooxygenases, are introduced into a suitable fungal host such as Aspergillus oryzae. This process results in the production of aphidicolan-16β-ol, which can be further converted to this compound .
Industrial Production Methods: Industrial production of this compound follows similar biosynthetic pathways, utilizing genetically engineered fungal strains to optimize yield and efficiency. The process involves fermentation under controlled conditions, followed by extraction and purification of the compound .
Chemical Reactions Analysis
Types of Reactions: 3-Deoxyaphidicolin undergoes various chemical reactions, including oxidation, reduction, and substitution. For instance, oxidation reactions can differentiate 1,3-glycols, leading to the formation of ketones .
Common Reagents and Conditions:
Oxidation: Sodium periodate is used to cleave glycols, resulting in ketones.
Reduction: Common reducing agents include lithium aluminum hydride.
Substitution: Halogenation reactions can be performed using reagents like thionyl chloride.
Major Products: The major products formed from these reactions include ketones, alcohols, and halogenated derivatives, depending on the specific reagents and conditions used .
Scientific Research Applications
Inhibition of DNA Polymerases
3-Deoxyaphidicolin is primarily recognized for its role as an inhibitor of DNA polymerase alpha. Studies have demonstrated that it possesses approximately one-third the activity of aphidicolin in inhibiting this enzyme, which is crucial for DNA replication.
Key Findings
- The compound has shown significant inhibition of in vivo DNA synthesis in cellular models, indicating its potential utility in experimental settings for studying DNA replication processes .
Cancer Research
The antitumor properties of this compound are notable, particularly due to its specificity for eukaryotic DNA polymerases. While aphidicolin has been tested in clinical trials, modifications like this compound may offer insights into developing more effective cancer therapies.
Case Studies
- In Vitro Studies : Research indicates that this compound has been effective against various human tumor cell lines, demonstrating growth-inhibitory effects that could be leveraged for therapeutic applications .
- Cell Cycle Synchronization : The compound can induce cell cycle arrest at the G1/S boundary, making it useful for synchronizing mammalian cell cultures in experimental setups .
Structure-Activity Relationship Studies
Understanding the structure-activity relationship (SAR) of this compound has been critical in elucidating its biological activity. Modifications to the hydroxyl groups on the aphidicolane ring have been systematically studied to assess their impact on inhibitory efficacy.
Research Insights
- Hydroxyl groups at positions 16 and 17 are essential for maintaining inhibitory activity against DNA polymerase alpha. In contrast, the absence of the 3a-hydroxyl group does not significantly diminish activity, suggesting that other structural features may compensate for this modification .
Comparative Data Table
The following table summarizes key findings related to the inhibitory activity of this compound compared to other derivatives:
Compound | IC50 (µg/ml) | Activity Level Compared to Aphidicolin |
---|---|---|
Aphidicolin | 0.44 | Baseline (100%) |
This compound | 0.89 | ~33% |
Aphidicolin-17-monoacetate | - | Inactive |
17-Acetylaphidicolin | - | ~10% weaker than aphidicolin |
Future Directions and Research Potential
The ongoing exploration of this compound's applications suggests several avenues for future research:
- Novel Therapeutics : Investigating modifications that enhance solubility while retaining inhibitory potency could lead to new cancer treatments.
- Mechanistic Studies : Further studies into the detailed mechanisms by which this compound interacts with DNA polymerases could provide insights into its selectivity and potential side effects.
Mechanism of Action
3-Deoxyaphidicolin exerts its effects by specifically inhibiting DNA polymerase α. This inhibition blocks the binding of deoxycytidine triphosphate at the active site of the enzyme, thereby halting DNA replication. The compound’s unique structure allows it to interact with the enzyme’s active site, preventing the incorporation of nucleotides into the growing DNA strand .
Comparison with Similar Compounds
Aphidicolin: The parent compound, known for its potent inhibition of DNA polymerase α.
Aphidicolan-16β-ol: An intermediate in the biosynthesis of aphidicolin and its derivatives.
Aphidicolin-17-monoacetate: A derivative with similar biological activities but different pharmacokinetic properties
Uniqueness: 3-Deoxyaphidicolin is unique due to its specific inhibition of DNA polymerase α and its distinct structural features. Unlike aphidicolin, it lacks certain hydroxyl groups, which may influence its interaction with the enzyme and its overall biological activity .
Biological Activity
3-Deoxyaphidicolin is a tetracyclic diterpenoid that has garnered attention due to its biological activity, particularly as an inhibitor of DNA polymerase α. This compound is a derivative of aphidicolin, which is known for its potent antimitotic and antiviral properties. This article reviews the biological activity of this compound, focusing on its mechanisms of action, efficacy against various biological targets, and potential therapeutic applications.
This compound functions primarily as a selective inhibitor of eukaryotic DNA polymerases, particularly DNA polymerase α. It acts through competitive inhibition with respect to deoxycytidine triphosphate (dCTP). Studies have demonstrated that this compound inhibits DNA synthesis in various cell types, including HeLa cells and sea urchin embryos, without affecting RNA or protein synthesis. The inhibition is characterized by a Ki value of approximately 0.44 µg/ml, indicating a strong affinity for the enzyme compared to other dNTPs .
Comparative Inhibition Data
Compound | Ki Value (µg/ml) | Target Enzyme |
---|---|---|
This compound | 0.44 | DNA Polymerase α |
Aphidicolin Monoacetate | 0.89 | DNA Polymerase α |
The specificity of this compound is notable; it does not inhibit DNA polymerases β and γ, which are involved in different cellular processes . This selective inhibition allows for targeted applications in research and potential therapeutic settings.
Antitumor Effects
Research indicates that this compound exhibits cytotoxic effects against various human tumor cell lines. The compound's ability to halt DNA replication makes it a candidate for further exploration in cancer therapy. However, like its parent compound aphidicolin, it faces challenges related to solubility and metabolic stability, which limit its clinical application .
Antiparasitic Activity
Recent studies have highlighted the leishmanicidal properties of this compound. It has shown significant activity against Leishmania species at concentrations as low as 0.19 µM without cytotoxic effects on mammalian cells. This suggests potential for development as an antiparasitic agent .
Case Studies and Research Findings
- Inhibition in Sea Urchin Embryos : In vivo studies demonstrated that treatment with this compound resulted in delayed cleavage of fertilized sea urchin eggs due to inhibited DNA synthesis, indicating its potent biological activity .
- Cytotoxicity in Human Tumor Cells : A study conducted on various human tumor cell lines revealed that this compound exhibited significant growth-inhibitory effects, reinforcing its potential utility in cancer treatment .
Structural Insights
The structural analysis of the interaction between this compound and DNA polymerase α provides insights into its inhibitory mechanism. The compound binds near the nucleotide-binding site, disrupting the incorporation of dCTP into the growing DNA strand. This structural understanding is crucial for the design of more effective derivatives with improved pharmacological profiles .
Q & A
Basic Research Questions
Q. What are the established methods for synthesizing 3-Deoxyaphidicolin in laboratory settings, and how do yield optimization strategies differ between fungal and yeast expression systems?
- Methodological Answer : The heterologous biosynthesis of this compound involves expressing a gene cluster (PbGGS, PbACS, PbP450-1, PbP450-2) in fungal hosts like Aspergillus oryzae or yeast (Saccharomyces cerevisiae). In A. oryzae, tetraploid transformants yield this compound, while diploid/trinuclear strains produce intermediates like aphidicolan-16β-ol . For yeast systems, precursor availability limits yields; thus, strategies include optimizing terpene synthase activity and enhancing geranylgeranyl diphosphate (GGPP) precursor supply via metabolic engineering (e.g., upregulating mevalonate pathway genes). Comparative studies should include LC-MS quantification of intermediates and final products across host systems .
Q. What experimental approaches are used to confirm this compound's specificity as a DNA polymerase α inhibitor, and how do researchers control for off-target effects in enzymatic assays?
- Methodological Answer : Specificity is validated via competitive inhibition assays using purified DNA polymerase α and structurally similar enzymes (e.g., DNA polymerase δ/ε). Researchers employ kinetic analyses (e.g., determination) and use negative controls with enzyme-active site mutations. To mitigate off-target effects, assays should include parallel testing with unrelated polymerases and use ATP-competitive inhibitors as reference compounds. Dose-response curves across multiple enzyme batches ensure reproducibility .
Q. How can researchers ensure reproducibility in chromatographic purification of this compound, and what analytical standards are recommended for purity validation?
- Methodological Answer : Reproducibility requires standardized protocols for HPLC/GC-MS conditions (e.g., C18 columns, isocratic elution with acetonitrile/water). Purity is validated using NMR (¹H/¹³C) and high-resolution mass spectrometry (HRMS). Internal standards (e.g., deuterated aphidicolin derivatives) and cross-referencing with published spectral data (JSTOR, PubMed Central) are critical. Document column lot numbers and solvent suppliers to address batch variability .
Advanced Research Questions
Q. What are the key challenges in achieving high-yield heterologous production of this compound in non-native fungal hosts, and how can metabolic engineering address rate-limiting steps in diterpene precursor supply?
- Methodological Answer : Challenges include inefficient cytochrome P450 activity and GGPP precursor limitations. Metabolic engineering solutions:
- Precursor enhancement : Overexpress GGPP synthase and downregulate competing pathways (e.g., ergosterol biosynthesis) via CRISPRi .
- Enzyme compatibility : Use codon-optimized P450 genes paired with redox partners (e.g., A. oryzae cytochrome P450 reductase) to improve hydroxylation efficiency .
- Dynamic regulation : Implement inducible promoters (e.g., amylase promoters) to stagger gene expression and reduce metabolic burden .
Q. How should researchers resolve contradictions in reported bioactivity data for this compound across different cell lines, and what statistical frameworks are recommended for cross-study comparability?
- Methodological Answer : Contradictions often arise from cell line-specific genetic backgrounds or assay conditions. Mitigation strategies:
- Standardization : Use authenticated cell lines (ATCC) and report doubling times/passage numbers .
- Meta-analysis : Apply random-effects models to aggregate data across studies, adjusting for variables like IC₅₀ normalization methods .
- Mechanistic follow-up : Perform transcriptomic profiling (RNA-seq) to identify cell line-specific resistance pathways (e.g., DNA repair gene upregulation) .
Q. What computational and experimental methods are used to elucidate the structure-activity relationship (SAR) of this compound analogs, and how can molecular docking inform iterative synthesis?
- Methodological Answer : SAR studies combine:
- Molecular docking : Simulate binding to DNA polymerase α’s active site (PDB ID: 1FVL) using AutoDock Vina; prioritize analogs with improved hydrogen-bonding to residues like Asp785 .
- Stereochemical analysis : Compare analogs’ activity via enantioselective synthesis and X-ray crystallography.
- In vitro validation : Test top candidates in polymerase inhibition assays and cytotoxicity screens (e.g., MTT assays on HEK293T vs. cancer cells) .
Q. How can researchers design robust control experiments to distinguish this compound’s direct enzymatic inhibition from indirect effects on cell cycle regulation?
- Methodological Answer : Use a tiered approach:
- In vitro : Compare inhibition kinetics of purified polymerase α vs. cell lysates.
- Genetic controls : Knock out polymerase α in cell lines (CRISPR/Cas9) and assess resistance.
- Cell cycle synchronization : Combine with thymidine-blocked cells to isolate G1/S phase effects .
Q. Methodological Best Practices
- Data Reporting : Follow the Beilstein Journal’s guidelines: include raw spectral data in supplementary materials, and cite original synthesis protocols to ensure reproducibility .
- Literature Review : Use scoping studies (Arksey & O’Malley framework) to map biosynthesis pathways and bioactivity data, prioritizing primary sources from PubMed and ACS Publications .
Properties
IUPAC Name |
(1S,2S,6R,7R,10S,12R,13R)-6,13-bis(hydroxymethyl)-2,6-dimethyltetracyclo[10.3.1.01,10.02,7]hexadecan-13-ol | |
---|---|---|
Source | PubChem | |
URL | https://pubchem.ncbi.nlm.nih.gov | |
Description | Data deposited in or computed by PubChem | |
InChI |
InChI=1S/C20H34O3/c1-17(12-21)6-3-7-18(2)16(17)5-4-14-10-15-11-19(14,18)8-9-20(15,23)13-22/h14-16,21-23H,3-13H2,1-2H3/t14-,15+,16-,17-,18-,19-,20-/m0/s1 | |
Source | PubChem | |
URL | https://pubchem.ncbi.nlm.nih.gov | |
Description | Data deposited in or computed by PubChem | |
InChI Key |
VHWTVINEPPOCLA-DTMQFJJTSA-N | |
Source | PubChem | |
URL | https://pubchem.ncbi.nlm.nih.gov | |
Description | Data deposited in or computed by PubChem | |
Canonical SMILES |
CC1(CCCC2(C1CCC3C24CCC(C(C3)C4)(CO)O)C)CO | |
Source | PubChem | |
URL | https://pubchem.ncbi.nlm.nih.gov | |
Description | Data deposited in or computed by PubChem | |
Isomeric SMILES |
C[C@]1(CCC[C@]2([C@H]1CC[C@@H]3[C@@]24CC[C@@]([C@H](C3)C4)(CO)O)C)CO | |
Source | PubChem | |
URL | https://pubchem.ncbi.nlm.nih.gov | |
Description | Data deposited in or computed by PubChem | |
Molecular Formula |
C20H34O3 | |
Source | PubChem | |
URL | https://pubchem.ncbi.nlm.nih.gov | |
Description | Data deposited in or computed by PubChem | |
Molecular Weight |
322.5 g/mol | |
Source | PubChem | |
URL | https://pubchem.ncbi.nlm.nih.gov | |
Description | Data deposited in or computed by PubChem | |
CAS No. |
85483-00-7 | |
Record name | 3-Deoxyaphidicolin | |
Source | ChemIDplus | |
URL | https://pubchem.ncbi.nlm.nih.gov/substance/?source=chemidplus&sourceid=0085483007 | |
Description | ChemIDplus is a free, web search system that provides access to the structure and nomenclature authority files used for the identification of chemical substances cited in National Library of Medicine (NLM) databases, including the TOXNET system. | |
Retrosynthesis Analysis
AI-Powered Synthesis Planning: Our tool employs the Template_relevance Pistachio, Template_relevance Bkms_metabolic, Template_relevance Pistachio_ringbreaker, Template_relevance Reaxys, Template_relevance Reaxys_biocatalysis model, leveraging a vast database of chemical reactions to predict feasible synthetic routes.
One-Step Synthesis Focus: Specifically designed for one-step synthesis, it provides concise and direct routes for your target compounds, streamlining the synthesis process.
Accurate Predictions: Utilizing the extensive PISTACHIO, BKMS_METABOLIC, PISTACHIO_RINGBREAKER, REAXYS, REAXYS_BIOCATALYSIS database, our tool offers high-accuracy predictions, reflecting the latest in chemical research and data.
Strategy Settings
Precursor scoring | Relevance Heuristic |
---|---|
Min. plausibility | 0.01 |
Model | Template_relevance |
Template Set | Pistachio/Bkms_metabolic/Pistachio_ringbreaker/Reaxys/Reaxys_biocatalysis |
Top-N result to add to graph | 6 |
Feasible Synthetic Routes
Disclaimer and Information on In-Vitro Research Products
Please be aware that all articles and product information presented on BenchChem are intended solely for informational purposes. The products available for purchase on BenchChem are specifically designed for in-vitro studies, which are conducted outside of living organisms. In-vitro studies, derived from the Latin term "in glass," involve experiments performed in controlled laboratory settings using cells or tissues. It is important to note that these products are not categorized as medicines or drugs, and they have not received approval from the FDA for the prevention, treatment, or cure of any medical condition, ailment, or disease. We must emphasize that any form of bodily introduction of these products into humans or animals is strictly prohibited by law. It is essential to adhere to these guidelines to ensure compliance with legal and ethical standards in research and experimentation.