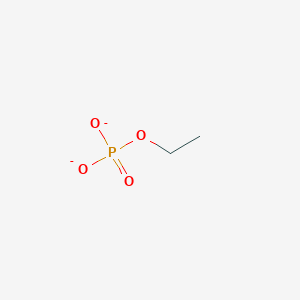
Ethylphosphate
Overview
Description
Ethyl phosphate(2-) is an organophosphate oxoanion that is the dianion of ethyl phosphate arising from deprotonation of both OH groups of the phosphate. It has a role as an epitope, a phosphoantigen and a metabolite. It is a conjugate base of an ethyl hydrogen phosphate(1-).
Scientific Research Applications
Pharmaceutical Applications
Drug Delivery Systems
One significant application of ethylphosphate is in the formulation of drug delivery systems. For example, bis(2-(methacryloyloxy)ethyl) phosphate has been utilized in creating microsphere formulations for controlled release of paclitaxel, a chemotherapeutic agent used in ovarian cancer treatment. These formulations are designed to deliver the drug over extended periods (up to eight weeks), enhancing therapeutic efficacy while minimizing side effects .
Case Study: Paclitaxel Microspheres
A study demonstrated that the incorporation of bis(2-(methacryloyloxy)ethyl) phosphate into paclitaxel microspheres improved the release profile and stability of the drug. The formulation was assessed for its effectiveness in inhibiting tumor growth in preclinical models, showing promising results that warrant further investigation in clinical settings .
Industrial Applications
Plasticizers and Flame Retardants
Triethyl phosphate serves as a plasticizer and flame retardant in various polymer systems. Its ability to enhance flexibility and durability makes it valuable in manufacturing unsaturated polyesters and other plastics. Additionally, it acts as a solvent for cellulose acetate and is used as an intermediate in the synthesis of pesticides .
Table 1: Industrial Applications of Triethyl Phosphate
Application | Description |
---|---|
Plasticizer | Improves flexibility and processability of polymers |
Flame Retardant | Reduces flammability of materials |
Solvent | Used in the dissolution of cellulose acetate |
Intermediate | Serves as a precursor in pesticide synthesis |
Environmental Applications
Biodegradable Composites
this compound derivatives have been explored for their role in developing biodegradable composites. Research indicates that ionic liquids assisted by this compound can facilitate the processing of renewable resources into eco-friendly materials, addressing environmental concerns associated with traditional plastics .
Dental Applications
Dental Adhesives
In dental applications, bis(2-(methacryloyloxy)ethyl) phosphate is utilized as a primer for enamel bonding systems. Its incorporation into dental adhesives enhances adhesion to tooth structure while providing antibacterial properties, which is crucial for long-term success in restorative dentistry .
Case Study: Dental Bonding Systems
A comparative study evaluated the performance of dental adhesives containing bis(2-(methacryloyloxy)ethyl) phosphate against traditional systems. Results indicated superior bonding strength and reduced hydrolysis susceptibility, making it a promising candidate for future adhesive formulations .
Summary of Findings
The applications of this compound are vast and varied, spanning pharmaceutical formulations, industrial processes, environmental sustainability efforts, and dental technologies. The following table summarizes key applications along with their benefits:
Application Area | Specific Use | Benefits |
---|---|---|
Pharmaceuticals | Drug delivery systems | Controlled release; enhanced stability |
Industrial | Plasticizers; flame retardants | Improved material properties |
Environmental | Biodegradable composites | Eco-friendly alternatives to traditional plastics |
Dental | Adhesives for enamel bonding | Enhanced adhesion; antibacterial properties |
Chemical Reactions Analysis
Acid-Catalyzed Hydrolysis
Ethyl phosphate undergoes acid-catalyzed hydrolysis via the BAL2 mechanism , involving bond cleavage between the phosphorus and oxygen atoms. The reaction proceeds through a trigonal bipyramidal intermediate, with the leaving group (ethanol) departing in the rate-determining step .
Table 1: Activation Parameters for Acid-Catalyzed Hydrolysis
Parameter | Value (kcal/mol) |
---|---|
ΔH‡ (activation enthalpy) | 24.6 |
ΔS‡ (activation entropy) | -18.0 |
Solvent | 35% Dioxane-65% Water |
The reaction rate increases with acid concentration, as protonation stabilizes the transition state .
Base-Catalyzed Hydrolysis
In alkaline conditions, ethyl phosphate hydrolyzes via an S_N2P mechanism , where a nucleophile (e.g., hydroxide ion) attacks the phosphorus center. This pathway is faster than acid-catalyzed hydrolysis due to the stronger nucleophilicity of the hydroxide ion .
Table 2: Rate Constants for Base-Catalyzed Hydrolysis
Nucleophile | Rate Constant (M⁻¹s⁻¹) |
---|---|
Hydroxide Ion | 1.2 × 10⁻³ |
Ammonia | 5.8 × 10⁻⁵ |
Nucleophilic Substitution Reactions
Ethyl phosphate reacts with α-effect nucleophiles (e.g., hydroxylamine, hydroperoxide) at accelerated rates due to stabilizing interactions between the nucleophile’s lone pairs and the phosphorus center.
Table 3: Reactivity of α-Effect Nucleophiles
Nucleophile | Rate Constant (M⁻¹s⁻¹) | Brønsted Slope (βₙᵤₙₙ) |
---|---|---|
Hydroxylamine | 2.1 × 10⁻² | 0.41 |
Hydroperoxide | 1.5 × 10⁻¹ | 0.38 |
The Brønsted correlation (βₙᵤₙₙ = 0.41) indicates strong electronic effects, with nucleophilicity correlating to basicity .
Enzymatic Hydrolysis
Ethyl phosphate is efficiently hydrolyzed by phosphatases, which utilize a two-metal ion mechanism . The enzyme active site coordinates the phosphate oxygen and facilitates nucleophilic attack by a metal-bound water molecule .
Table 4: Enzymatic Hydrolysis Parameters
Enzyme | K_M (mM) | k_cat (s⁻¹) |
---|---|---|
Alkaline Phosphatase | 0.35 | 120 |
This mechanism achieves rate accelerations exceeding 10⁷-fold compared to uncatalyzed reactions .
Environmental Considerations
Table 5: Biodegradation Pathways
Degradant | Half-Life (days) |
---|---|
Ethyl Dihydrogen Phosphate | 5–7 |
Inorganic Phosphate | Stable |
Q & A
Basic Research Questions
Q. What are the established protocols for synthesizing ethylphosphate derivatives with high purity?
- Methodology : this compound synthesis typically involves esterification of phosphoric acid with ethanol under controlled anhydrous conditions. Key steps include:
- Use of catalysts (e.g., sulfuric acid) to accelerate ester bond formation.
- Purification via fractional distillation or recrystallization, monitored by nuclear magnetic resonance (NMR) or high-performance liquid chromatography (HPLC) .
- Analytical validation through mass spectrometry (MS) to confirm molecular weight (e.g., 275.22 g/mol for diethyl-p-nitrophenyl phosphate) .
Q. How can researchers detect and quantify this compound metabolites in biological samples?
- Methodology :
- Extraction : Liquid-liquid extraction using organic solvents (e.g., ethyl acetate) to isolate this compound derivatives from urine or plasma.
- Detection : Gas chromatography-mass spectrometry (GC-MS) or liquid chromatography-tandem MS (LC-MS/MS) with isotopic internal standards (e.g., deuterated analogs) .
- Validation : Calibration curves spanning 1–500 ng/mL, with recovery rates >85% and limits of detection (LOD) <1 ng/mL .
Q. What mechanisms underlie this compound toxicity in acetylcholinesterase inhibition?
- Mechanistic Insight : this compound derivatives (e.g., paraoxon) phosphorylate serine residues in acetylcholinesterase’s catalytic triad, irreversibly blocking neurotransmitter hydrolysis.
- Experimental Validation :
- In vitro assays with purified enzyme and spectrophotometric monitoring of substrate (acetylthiocholine) depletion .
- Dose-response curves to calculate IC₅₀ values, cross-referenced with molecular docking simulations .
Advanced Research Questions
Q. How do computational models resolve contradictions in this compound reaction kinetics across experimental studies?
- Methodology :
-
Quantum Mechanics/Molecular Mechanics (QM/MM) : Simulate transition states of this compound hydrolysis to identify rate-limiting steps (e.g., nucleophilic attack vs. leaving group departure) .
-
Kinetic Isotope Effects (KIEs) : Compare experimental ¹⁸O KIEs (1.039–1.001) with computational predictions (1.046) to validate stepwise vs. concerted mechanisms (Table 1) .
Table 1 : Kinetic Isotope Effects (KIEs) for this compound Hydrolysis
Parameter Experimental KIE Computational KIE Nucleophile (O2′) 1.010 0.998–0.981 Leaving Group (O5′) 1.041 1.046 Source: Adapted from
Q. What strategies reconcile discrepancies between in vitro and in vivo toxicity data for this compound compounds?
- Methodology :
- Toxicokinetic Modeling : Incorporate species-specific metabolic rates (e.g., hepatic cytochrome P450 activity) to adjust NOEL (no-observed-effect-level) values. For example, oral biological reference values for this compound metabolites in children are 52 nmol/kg .
- Cross-Species Extrapolation : Use allometric scaling to convert rodent toxicity data to human equivalents .
Q. How can metabolomics data improve risk assessment of this compound exposure in vulnerable populations?
- Methodology :
- Biomonitoring : Measure urinary this compound metabolites (e.g., diethylthiophosphate) in longitudinal cohort studies.
- Statistical Analysis : Apply multivariate regression to correlate metabolite levels with neurodevelopmental outcomes, adjusting for covariates (e.g., age, diet) .
Q. Data Contradiction Analysis
- Case Study : Variability in reported KIEs for this compound hydrolysis (Table 1) arises from differences in solvent systems (aqueous vs. nonpolar) and pH conditions. Researchers should standardize reaction parameters and validate findings using dual experimental-computational approaches .
Preparation Methods
Catalytic Rearrangement Using Ethyl p-Toluenesulfonate
The catalytic rearrangement of triethyl phosphite using ethyl p-toluenesulfonate represents a high-yield industrial method for synthesizing diethyl ethylphosphate. As detailed in CN101863920B, this process involves refluxing triethyl phosphite with 0.3–1% (by mass) ethyl p-toluenesulfonate at 180–185°C for 3–4 hours, followed by vacuum distillation to isolate the product at 118–120°C under 0.095 MPa . The catalyst remains active in the residual liquid, enabling reuse across multiple batches without significant efficiency loss.
Reaction Mechanism and Optimization
The reaction proceeds via a Michaelis-Arbuzov-like rearrangement, where the catalyst facilitates nucleophilic substitution at the phosphorus center. Key advantages include:
-
Yield : 98% or higher, attributed to precise temperature control and catalytic efficiency .
-
Purity : The distillate is colorless and transparent, with a boiling point of 198–199°C, indicating minimal byproducts .
-
Environmental Impact : No halogenated byproducts are generated, addressing toxicity concerns associated with traditional haloalkane catalysts .
Table 1: Parameters for Catalytic Rearrangement
Parameter | Value/Range |
---|---|
Reactant | Triethyl phosphite |
Catalyst | Ethyl p-toluenesulfonate |
Catalyst Loading | 0.3–1% (w/w) |
Reaction Temperature | 180–185°C |
Distillation Conditions | 118–120°C, 0.095 MPa |
Yield | ≥98% |
Solvent-Free Synthesis from Triethyl Phosphite and Phosphorous Acid
A solvent- and catalyst-free method for synthesizing diethyl phosphite, a precursor to this compound derivatives, is described in CN111995639A. This approach involves the batch addition of phosphorous acid to triethyl phosphite under controlled thermal conditions .
Stepwise Process
-
Batch Addition : Phosphorous acid is added in 4–5 increments to triethyl phosphite at ≤35°C to mitigate exothermic effects .
-
Thermal Reaction : The mixture is held at 50–60°C for 30 minutes, then gradually heated to 60–90°C at 2.5–5°C/h for 3–5 hours .
-
Product Isolation : Diethyl phosphite is obtained with 97–99% yield and ≥99% purity, confirmed by gas chromatography .
Table 2: Solvent-Free Synthesis Parameters
Parameter | Value/Range |
---|---|
Reactants | Triethyl phosphite, H₃PO₃ |
Phosphorous Acid Addition | 4–5 batches |
Initial Temperature | ≤35°C |
Final Temperature | 60–90°C |
Reaction Time | 3–5 hours |
Yield | 97–99% |
This method eliminates solvent waste and reduces energy consumption compared to traditional routes, making it suitable for scalable production .
Eco-Friendly Synthesis with Phosphoric Acid and Ethanol
An environmentally benign synthesis of monoethyl phosphate (EtP) involves reacting phosphoric acid with absolute ethanol at room temperature . This method, optimized for flame-retardant applications, produces a mixture of mono- and diethyl phosphates in a 2:3 molar ratio .
Characterization and Applications
-
FTIR Analysis : Peaks at 805 cm⁻¹ (P–O–C), 1037 cm⁻¹ (P–O), and 1223 cm⁻¹ (P=O) confirm ester formation .
-
Mass Spectrometry : Fragments at m/z 99 (phosphoric acid), 127 (monoethyl phosphate), and 155 (diethyl phosphate) validate product identity .
-
Melamine Salt Derivative : EtP reacts with melamine to form a fire-retardant salt, demonstrating its utility in material science .
Biological Formation via In Vivo Alcoholysis
Monoethyl phosphate has been isolated from the livers of ethanol-intoxicated rats, revealing a biological synthesis pathway . Administering [2-¹⁴C]ethanol or [³²P]orthophosphate led to labeled this compound, confirmed via enzymatic hydrolysis and chromatographic analysis .
Mechanism and Implications
-
Alcoholysis : Ethanol substitutes water in hydrolytic enzyme-catalyzed reactions, yielding this compound from endogenous phosphate esters .
-
Physiological Impact : This pathway underscores the metabolic consequences of acute ethanol exposure, linking intoxication to aberrant phosphorylation .
Comparative Analysis of Preparation Methods
Table 3: Method Comparison
Method | Yield | Temperature | Catalysts/Solvents | Environmental Impact |
---|---|---|---|---|
Catalytic Rearrangement | ≥98% | 180–185°C | Ethyl p-toluenesulfonate | Low toxicity |
Solvent-Free Synthesis | 97–99% | 60–90°C | None | Solvent-free |
Eco-Friendly Synthesis | 98% | Room temp. | None | Low energy input |
Biological Formation | Trace | 37°C (in vivo) | Enzymatic | N/A |
Key Considerations
-
Industrial Scalability : The catalytic and solvent-free methods are best suited for large-scale production due to high yields and simplicity .
-
Purity Requirements : Solvent-free synthesis achieves ≥99% purity, critical for pharmaceutical applications .
-
Biological Relevance : In vivo formation highlights potential toxicological risks of ethanol metabolism .
Properties
Molecular Formula |
C2H5O4P-2 |
---|---|
Molecular Weight |
124.03 g/mol |
IUPAC Name |
ethyl phosphate |
InChI |
InChI=1S/C2H7O4P/c1-2-6-7(3,4)5/h2H2,1H3,(H2,3,4,5)/p-2 |
InChI Key |
ZJXZSIYSNXKHEA-UHFFFAOYSA-L |
SMILES |
CCOP(=O)([O-])[O-] |
Canonical SMILES |
CCOP(=O)([O-])[O-] |
Origin of Product |
United States |
Retrosynthesis Analysis
AI-Powered Synthesis Planning: Our tool employs the Template_relevance Pistachio, Template_relevance Bkms_metabolic, Template_relevance Pistachio_ringbreaker, Template_relevance Reaxys, Template_relevance Reaxys_biocatalysis model, leveraging a vast database of chemical reactions to predict feasible synthetic routes.
One-Step Synthesis Focus: Specifically designed for one-step synthesis, it provides concise and direct routes for your target compounds, streamlining the synthesis process.
Accurate Predictions: Utilizing the extensive PISTACHIO, BKMS_METABOLIC, PISTACHIO_RINGBREAKER, REAXYS, REAXYS_BIOCATALYSIS database, our tool offers high-accuracy predictions, reflecting the latest in chemical research and data.
Strategy Settings
Precursor scoring | Relevance Heuristic |
---|---|
Min. plausibility | 0.01 |
Model | Template_relevance |
Template Set | Pistachio/Bkms_metabolic/Pistachio_ringbreaker/Reaxys/Reaxys_biocatalysis |
Top-N result to add to graph | 6 |
Feasible Synthetic Routes
Disclaimer and Information on In-Vitro Research Products
Please be aware that all articles and product information presented on BenchChem are intended solely for informational purposes. The products available for purchase on BenchChem are specifically designed for in-vitro studies, which are conducted outside of living organisms. In-vitro studies, derived from the Latin term "in glass," involve experiments performed in controlled laboratory settings using cells or tissues. It is important to note that these products are not categorized as medicines or drugs, and they have not received approval from the FDA for the prevention, treatment, or cure of any medical condition, ailment, or disease. We must emphasize that any form of bodily introduction of these products into humans or animals is strictly prohibited by law. It is essential to adhere to these guidelines to ensure compliance with legal and ethical standards in research and experimentation.