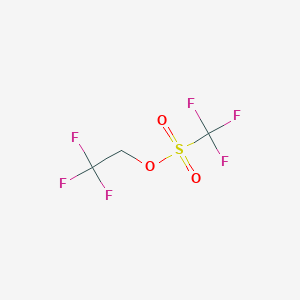
2,2,2-Trifluoroethyl trifluoromethanesulfonate
Overview
Description
2,2,2-Trifluoroethyl trifluoromethanesulfonate is a chemical compound with the molecular formula C3H2F6O3S. It is a powerful trifluoroethylating agent, which is useful for the synthesis of fluorinated amino acids and other fluorinated compounds .
Mechanism of Action
Target of Action
2,2,2-Trifluoroethyl trifluoromethanesulfonate is a powerful trifluoroethylating agent . It is primarily used in the synthesis of fluorinated amino acids . The primary targets of this compound are therefore the amino acids that are being fluorinated.
Mode of Action
The compound acts as a reagent in the enantioselective preparation of cyclic N-aryl hydroxamic acids via phase-transfer catalyzed alkylation of nitrobenzyl bromides . This results in the production of nitrophenylalanines .
Biochemical Pathways
The biochemical pathways affected by this compound are those involved in the synthesis of fluorinated amino acids . The downstream effects of this process include the production of fluorinated amino acids, which can have various applications in medicinal chemistry and drug design.
Result of Action
The result of the action of this compound is the production of fluorinated amino acids . These compounds can have various biological activities, depending on their specific structures and the nature of the fluorinated substituents.
Action Environment
The action of this compound can be influenced by various environmental factors. For example, the compound is sensitive to moisture . Therefore, it should be stored under dry conditions to maintain its stability and efficacy . Furthermore, the compound is a liquid that is slightly soluble in water , which can affect its distribution and reactivity in aqueous environments.
Biochemical Analysis
Biochemical Properties
It is known to be a powerful trifluoroethylating agent, which suggests that it may interact with various enzymes, proteins, and other biomolecules in biochemical reactions .
Molecular Mechanism
As a trifluoroethylating agent, it may exert its effects at the molecular level through binding interactions with biomolecules, potentially leading to changes in gene expression .
Preparation Methods
2,2,2-Trifluoroethyl trifluoromethanesulfonate can be synthesized through several methods. One common method involves the reaction of trifluoromethanesulfonic anhydride with 2,2,2-trifluoroethanol. The reaction is typically carried out in a nitrogen atmosphere at room temperature, followed by reflux for several hours . Another method involves the acylation of 2,2,2-trifluoroethanol with trifluoromethanesulfonyl chloride in the presence of a base such as triethylamine . The reaction mixture is cooled to -25°C and then allowed to warm to room temperature while stirring overnight .
Chemical Reactions Analysis
2,2,2-Trifluoroethyl trifluoromethanesulfonate undergoes various chemical reactions, including substitution reactions. It is commonly used as a reagent in the enantioselective preparation of cyclic N-aryl hydroxamic acids via phase-transfer catalyzed alkylation of nitrobenzyl bromides . The compound is also used in the synthesis of fluorinated amino acids . Major products formed from these reactions include nirophenylalanines and other fluorinated compounds .
Scientific Research Applications
2,2,2-Trifluoroethyl trifluoromethanesulfonate has several scientific research applications. It is widely used in organic synthesis as a powerful trifluoroethylating agent . In chemistry, it is used for the synthesis of fluorinated amino acids and other fluorinated compounds . In biology and medicine, it is used in the preparation of cyclic N-aryl hydroxamic acids, which have potential therapeutic applications . The compound is also used in the industry for the synthesis of various fluorinated materials .
Comparison with Similar Compounds
2,2,2-Trifluoroethyl trifluoromethanesulfonate is similar to other trifluoromethanesulfonates, such as methyl trifluoromethanesulfonate and ethyl trifluoromethanesulfonate . it is unique in its ability to introduce the trifluoroethyl group into target molecules, making it a valuable reagent in organic synthesis . Other similar compounds include phenyl trifluoromethanesulfonate and difluoromethyl triflate .
Biological Activity
2,2,2-Trifluoroethyl trifluoromethanesulfonate (CF₃SO₂OCH₂CF₃) is a synthetic organofluorine compound that has garnered attention for its unique chemical properties and potential biological activities. This article explores its biological activity, including its synthesis, mechanisms of action, and relevant case studies.
- Chemical Formula : C₃H₂F₆O₃S
- Molecular Weight : 232.10 g/mol
- CAS Number : 6226-25-1
- Physical State : Exists in gaseous, liquid, and solid states under different conditions .
Synthesis
The synthesis of this compound typically involves the reaction of trifluoromethanesulfonic acid with 2,2,2-trifluoroethyl alcohol. The following general reaction scheme illustrates this process:
Research indicates that this compound exhibits various biological activities, primarily related to its ability to modify cellular processes. Notably:
- Antiplasmodial Activity : A study demonstrated that derivatives of this compound show significant antiplasmodial activity against Plasmodium falciparum, the causative agent of malaria. The synthesized compounds were evaluated for their ability to inhibit the growth of the parasite in vitro .
- Differentiation Induction in Cancer Cells : In a study involving acute myeloid leukemia (AML) cells, this compound was shown to induce differentiation by upregulating CD11b expression and decreasing cell proliferation. This suggests a potential therapeutic role in hematological malignancies .
Case Studies and Research Findings
Safety and Toxicity
The compound is classified as hazardous:
Properties
IUPAC Name |
2,2,2-trifluoroethyl trifluoromethanesulfonate | |
---|---|---|
Source | PubChem | |
URL | https://pubchem.ncbi.nlm.nih.gov | |
Description | Data deposited in or computed by PubChem | |
InChI |
InChI=1S/C3H2F6O3S/c4-2(5,6)1-12-13(10,11)3(7,8)9/h1H2 | |
Source | PubChem | |
URL | https://pubchem.ncbi.nlm.nih.gov | |
Description | Data deposited in or computed by PubChem | |
InChI Key |
RTMMSCJWQYWMNK-UHFFFAOYSA-N | |
Source | PubChem | |
URL | https://pubchem.ncbi.nlm.nih.gov | |
Description | Data deposited in or computed by PubChem | |
Canonical SMILES |
C(C(F)(F)F)OS(=O)(=O)C(F)(F)F | |
Source | PubChem | |
URL | https://pubchem.ncbi.nlm.nih.gov | |
Description | Data deposited in or computed by PubChem | |
Molecular Formula |
C3H2F6O3S | |
Source | PubChem | |
URL | https://pubchem.ncbi.nlm.nih.gov | |
Description | Data deposited in or computed by PubChem | |
DSSTOX Substance ID |
DTXSID1073365 | |
Record name | 2,2,2-Trifluoroethyl triflate | |
Source | EPA DSSTox | |
URL | https://comptox.epa.gov/dashboard/DTXSID1073365 | |
Description | DSSTox provides a high quality public chemistry resource for supporting improved predictive toxicology. | |
Molecular Weight |
232.10 g/mol | |
Source | PubChem | |
URL | https://pubchem.ncbi.nlm.nih.gov | |
Description | Data deposited in or computed by PubChem | |
CAS No. |
6226-25-1 | |
Record name | 2,2,2-Trifluoroethyl trifluoromethanesulfonate | |
Source | CAS Common Chemistry | |
URL | https://commonchemistry.cas.org/detail?cas_rn=6226-25-1 | |
Description | CAS Common Chemistry is an open community resource for accessing chemical information. Nearly 500,000 chemical substances from CAS REGISTRY cover areas of community interest, including common and frequently regulated chemicals, and those relevant to high school and undergraduate chemistry classes. This chemical information, curated by our expert scientists, is provided in alignment with our mission as a division of the American Chemical Society. | |
Explanation | The data from CAS Common Chemistry is provided under a CC-BY-NC 4.0 license, unless otherwise stated. | |
Record name | Methanesulfonic acid, trifluoro-, 2,2,2-trifluoroethyl ester | |
Source | ChemIDplus | |
URL | https://pubchem.ncbi.nlm.nih.gov/substance/?source=chemidplus&sourceid=0006226251 | |
Description | ChemIDplus is a free, web search system that provides access to the structure and nomenclature authority files used for the identification of chemical substances cited in National Library of Medicine (NLM) databases, including the TOXNET system. | |
Record name | 2,2,2-Trifluoroethyl triflate | |
Source | EPA DSSTox | |
URL | https://comptox.epa.gov/dashboard/DTXSID1073365 | |
Description | DSSTox provides a high quality public chemistry resource for supporting improved predictive toxicology. | |
Record name | 2,2,2-Trifluoroethyl Trifluoromethanesulfonate | |
Source | European Chemicals Agency (ECHA) | |
URL | https://echa.europa.eu/substance-information/-/substanceinfo/100.104.621 | |
Description | The European Chemicals Agency (ECHA) is an agency of the European Union which is the driving force among regulatory authorities in implementing the EU's groundbreaking chemicals legislation for the benefit of human health and the environment as well as for innovation and competitiveness. | |
Explanation | Use of the information, documents and data from the ECHA website is subject to the terms and conditions of this Legal Notice, and subject to other binding limitations provided for under applicable law, the information, documents and data made available on the ECHA website may be reproduced, distributed and/or used, totally or in part, for non-commercial purposes provided that ECHA is acknowledged as the source: "Source: European Chemicals Agency, http://echa.europa.eu/". Such acknowledgement must be included in each copy of the material. ECHA permits and encourages organisations and individuals to create links to the ECHA website under the following cumulative conditions: Links can only be made to webpages that provide a link to the Legal Notice page. | |
Record name | Methanesulfonic acid, 1,1,1-trifluoro-, 2,2,2-trifluoroethyl ester | |
Source | European Chemicals Agency (ECHA) | |
URL | https://echa.europa.eu/substance-information/-/substanceinfo/100.120.718 | |
Description | The European Chemicals Agency (ECHA) is an agency of the European Union which is the driving force among regulatory authorities in implementing the EU's groundbreaking chemicals legislation for the benefit of human health and the environment as well as for innovation and competitiveness. | |
Explanation | Use of the information, documents and data from the ECHA website is subject to the terms and conditions of this Legal Notice, and subject to other binding limitations provided for under applicable law, the information, documents and data made available on the ECHA website may be reproduced, distributed and/or used, totally or in part, for non-commercial purposes provided that ECHA is acknowledged as the source: "Source: European Chemicals Agency, http://echa.europa.eu/". Such acknowledgement must be included in each copy of the material. ECHA permits and encourages organisations and individuals to create links to the ECHA website under the following cumulative conditions: Links can only be made to webpages that provide a link to the Legal Notice page. | |
Synthesis routes and methods I
Procedure details
Synthesis routes and methods II
Procedure details
Synthesis routes and methods III
Procedure details
Retrosynthesis Analysis
AI-Powered Synthesis Planning: Our tool employs the Template_relevance Pistachio, Template_relevance Bkms_metabolic, Template_relevance Pistachio_ringbreaker, Template_relevance Reaxys, Template_relevance Reaxys_biocatalysis model, leveraging a vast database of chemical reactions to predict feasible synthetic routes.
One-Step Synthesis Focus: Specifically designed for one-step synthesis, it provides concise and direct routes for your target compounds, streamlining the synthesis process.
Accurate Predictions: Utilizing the extensive PISTACHIO, BKMS_METABOLIC, PISTACHIO_RINGBREAKER, REAXYS, REAXYS_BIOCATALYSIS database, our tool offers high-accuracy predictions, reflecting the latest in chemical research and data.
Strategy Settings
Precursor scoring | Relevance Heuristic |
---|---|
Min. plausibility | 0.01 |
Model | Template_relevance |
Template Set | Pistachio/Bkms_metabolic/Pistachio_ringbreaker/Reaxys/Reaxys_biocatalysis |
Top-N result to add to graph | 6 |
Feasible Synthetic Routes
Q1: What is the significance of the reaction between 2,2,2-trifluoroethyl trifluoromethanesulfonate and p-dimethylaminophenylmagnesium bromide?
A1: This reaction, as explored in one study , investigates the reactivity of perfluoroalkanesulfonate esters with Grignard reagents. While the specific findings aren't detailed in the abstract, this type of reaction generally explores the potential for nucleophilic substitution reactions on the alkyl chain of the sulfonate ester. Understanding such reactions can provide insights into new synthetic pathways for incorporating fluoroalkyl groups into organic molecules.
Q2: How does the structure of this compound influence its reactivity as a fluorinating agent?
A2: The molecule consists of a trifluoromethanesulfonate group (CF3SO2O-), a highly electron-withdrawing group, attached to a 2,2,2-trifluoroethyl group (CF3CH2-). This structure makes the molecule a strong electrophile. The trifluoromethanesulfonate acts as an excellent leaving group, facilitating nucleophilic attack on the carbon adjacent to the fluorine atoms. This allows for the introduction of the 2,2,2-trifluoroethyl group into other molecules, serving as a valuable tool in medicinal and agricultural chemistry for modifying the properties of target compounds .
Q3: What are the potential applications of synthesizing 1-H-2,2-difluorovinylphosphorus compounds from this compound?
A3: While the specific applications aren't detailed in the provided abstract , 1-H-2,2-difluorovinylphosphorus compounds represent a class of organofluorine compounds with potential applications in various fields. These could include:
Q4: Are there any spectroscopic studies available on this compound?
A4: Yes, one study focuses on the experimental and theoretical vibrational study of this compound . Although the specific findings are not elaborated on in the abstract, this type of study likely provides insights into the molecule's structure and bonding characteristics. Vibrational spectroscopy techniques like infrared and Raman spectroscopy can be used to identify specific functional groups and analyze molecular vibrations, contributing to a more comprehensive understanding of the compound's properties.
Disclaimer and Information on In-Vitro Research Products
Please be aware that all articles and product information presented on BenchChem are intended solely for informational purposes. The products available for purchase on BenchChem are specifically designed for in-vitro studies, which are conducted outside of living organisms. In-vitro studies, derived from the Latin term "in glass," involve experiments performed in controlled laboratory settings using cells or tissues. It is important to note that these products are not categorized as medicines or drugs, and they have not received approval from the FDA for the prevention, treatment, or cure of any medical condition, ailment, or disease. We must emphasize that any form of bodily introduction of these products into humans or animals is strictly prohibited by law. It is essential to adhere to these guidelines to ensure compliance with legal and ethical standards in research and experimentation.