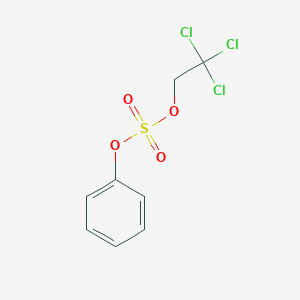
Phenyl 2,2,2-trichloroethyl sulfate
- Click on QUICK INQUIRY to receive a quote from our team of experts.
- With the quality product at a COMPETITIVE price, you can focus more on your research.
Overview
Description
Phenyl 2,2,2-trichloroethyl sulfate is an organic compound that belongs to the class of sulfate esters It is characterized by the presence of a phenyl group attached to a 2,2,2-trichloroethyl sulfate moiety
Preparation Methods
Synthetic Routes and Reaction Conditions
Phenyl 2,2,2-trichloroethyl sulfate can be synthesized through the sulfation of phenolic compounds using 2,2,2-trichloroethyl chlorosulfate. The reaction typically involves the use of a base such as N,N-dimethylaminopyridine (DMAP) to facilitate the sulfation process . The reaction conditions often include the use of an organic solvent and controlled temperature to ensure the desired product is obtained in good yield.
Industrial Production Methods
In an industrial setting, the production of this compound may involve large-scale sulfation processes using chlorosulfonic acid esters or sulfuryl imidazolium salts. These methods allow for milder reaction conditions and efficient production of the compound .
Chemical Reactions Analysis
Types of Reactions
Phenyl 2,2,2-trichloroethyl sulfate undergoes various chemical reactions, including:
Oxidation: The compound can be oxidized under specific conditions to form different oxidation products.
Reduction: Reduction reactions can lead to the formation of phenolic derivatives.
Substitution: The sulfate group can be substituted with other functional groups under appropriate conditions.
Common Reagents and Conditions
Common reagents used in the reactions of this compound include oxidizing agents, reducing agents, and nucleophiles. The reaction conditions vary depending on the desired transformation but often involve controlled temperature and the use of solvents such as methanol .
Major Products Formed
The major products formed from the reactions of this compound include phenolic derivatives, sulfated metabolites, and other substituted compounds .
Scientific Research Applications
Phenyl 2,2,2-trichloroethyl sulfate has several scientific research applications, including:
Mechanism of Action
The mechanism of action of phenyl 2,2,2-trichloroethyl sulfate involves its interaction with molecular targets such as enzymes and proteins. The compound can inhibit the activity of certain enzymes by binding to their active sites, thereby affecting their function. The pathways involved in its mechanism of action include the inhibition of sulfatases and other related enzymes .
Comparison with Similar Compounds
Phenyl 2,2,2-trichloroethyl sulfate can be compared with other similar compounds, such as:
4’-Chlorobiphenyl-3-yl 2,2,2-trichloroethyl sulfate: This compound has a similar sulfate ester structure but with a chlorinated biphenyl group.
Polychlorinated biphenyl sulfate derivatives: These compounds share the sulfate ester functionality but differ in their aromatic ring structures and degree of chlorination.
This compound is unique due to its specific phenyl and 2,2,2-trichloroethyl sulfate moiety, which imparts distinct chemical properties and reactivity compared to other sulfate esters.
Properties
CAS No. |
653605-14-2 |
---|---|
Molecular Formula |
C8H7Cl3O4S |
Molecular Weight |
305.6 g/mol |
IUPAC Name |
phenyl 2,2,2-trichloroethyl sulfate |
InChI |
InChI=1S/C8H7Cl3O4S/c9-8(10,11)6-14-16(12,13)15-7-4-2-1-3-5-7/h1-5H,6H2 |
InChI Key |
UAGOTNIBMAOPEM-UHFFFAOYSA-N |
Canonical SMILES |
C1=CC=C(C=C1)OS(=O)(=O)OCC(Cl)(Cl)Cl |
Origin of Product |
United States |
Disclaimer and Information on In-Vitro Research Products
Please be aware that all articles and product information presented on BenchChem are intended solely for informational purposes. The products available for purchase on BenchChem are specifically designed for in-vitro studies, which are conducted outside of living organisms. In-vitro studies, derived from the Latin term "in glass," involve experiments performed in controlled laboratory settings using cells or tissues. It is important to note that these products are not categorized as medicines or drugs, and they have not received approval from the FDA for the prevention, treatment, or cure of any medical condition, ailment, or disease. We must emphasize that any form of bodily introduction of these products into humans or animals is strictly prohibited by law. It is essential to adhere to these guidelines to ensure compliance with legal and ethical standards in research and experimentation.