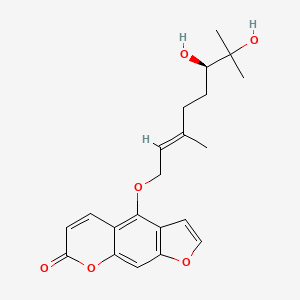
Dihydroxybergamottin
Overview
Description
Dihydroxybergamottin (DHB), chemically identified as 6',7'-dihydroxybergamottin, is a linear furanocoumarin predominantly found in grapefruit (Citrus paradisi) and its hybrids. It is synthesized via O-geranylation and subsequent epoxidation of bergaptol, a precursor coumarin . DHB is renowned for its role in the "grapefruit juice effect," where it inhibits cytochrome P450 3A4 (CYP3A4), a key enzyme in drug metabolism, thereby increasing the bioavailability of co-administered medications such as cyclosporine, statins, and calcium channel blockers . DHB also modulates P-glycoprotein (P-gp), an efflux transporter, though its primary mechanism involves mechanism-based inactivation of CYP3A4, leading to enzyme degradation in enterocytes .
DHB’s concentration in grapefruit juice varies by cultivar and processing methods, typically ranging from 1–30 µM, which exceeds its IC₅₀ (1 µM) for CYP3A4 inhibition . Unlike orange juice, which lacks DHB, grapefruit juice’s inhibitory potency correlates strongly with DHB content . DHB is detectable in human plasma and urine post-ingestion, underscoring its systemic bioavailability and clinical relevance .
Preparation Methods
Synthetic Routes and Reaction Conditions: Dihydroxybergamottin can be synthesized through the alkylation of umbelliferone with geraniol derivatives . The reaction typically involves the use of a base such as potassium carbonate in a solvent like dimethylformamide. The product is then purified through column chromatography.
Industrial Production Methods: Industrial production of this compound often involves extraction from natural sources, such as grapefruit juice. The extraction process includes steps like solvent extraction, filtration, and purification using techniques like high-performance liquid chromatography .
Types of Reactions:
Oxidation: this compound can undergo oxidation reactions, often catalyzed by enzymes like cytochrome P450.
Reduction: It can also participate in reduction reactions, although these are less common.
Substitution: The compound can undergo nucleophilic substitution reactions, particularly at the furan ring.
Common Reagents and Conditions:
Oxidation: Common oxidizing agents include hydrogen peroxide and molecular oxygen in the presence of a catalyst.
Reduction: Reducing agents like sodium borohydride can be used.
Substitution: Nucleophiles such as amines or thiols can be used under basic conditions.
Major Products: The major products of these reactions depend on the specific conditions and reagents used. For example, oxidation can lead to the formation of epoxides or hydroxylated derivatives .
Scientific Research Applications
Cytochrome P450 Inhibition
Mechanism of Action
Dihydroxybergamottin functions as a potent inhibitor of the CYP3A4 enzyme, which plays a crucial role in the metabolism of many drugs. The inhibition occurs through a mechanism-based (suicide) inhibition, where this compound binds irreversibly to the enzyme, reducing its activity significantly .
Pharmacokinetic Interactions
Research indicates that this compound can enhance the bioavailability of co-administered drugs metabolized by CYP3A4. For example, studies have shown that when felodipine is taken with grapefruit juice containing this compound, there is a notable increase in the drug's plasma concentration, enhancing its therapeutic effects .
Drug | Effect of this compound |
---|---|
Felodipine | Increased area under the curve (AUC) by 1.9-fold |
Midazolam | Enhanced bioavailability due to CYP3A4 inhibition |
Clinical Implications
Drug Formulation
The incorporation of this compound into drug formulations has potential benefits in improving the efficacy of certain medications by increasing their bioavailability. For instance, combining this compound with drugs like statins or calcium channel blockers could lead to more effective treatment regimens .
Case Studies
- A study involving healthy volunteers demonstrated that co-administration of grapefruit juice (rich in this compound) with felodipine resulted in significant pharmacokinetic changes, highlighting its role in enhancing drug absorption and effect .
- Another investigation assessed the impact of this compound on patients taking various medications that undergo CYP3A4 metabolism, revealing alterations in drug plasma levels and therapeutic outcomes .
Potential Therapeutic Uses
Anti-inflammatory and Antioxidative Properties
Recent studies suggest that this compound exhibits anti-inflammatory and antioxidative effects. These properties may contribute to its potential use in treating conditions characterized by oxidative stress and inflammation .
Property | Potential Application |
---|---|
Antioxidative | Management of oxidative stress-related diseases |
Anti-inflammatory | Treatment of inflammatory conditions |
Research Challenges and Future Directions
Despite promising findings regarding the applications of this compound, challenges remain in fully understanding its pharmacokinetics and interactions with other drugs. Future research should focus on:
- Detailed studies on the long-term effects of this compound in various populations.
- Exploration of its interactions with a broader range of pharmaceuticals.
- Development of standardized formulations that incorporate this compound to maximize therapeutic benefits.
Mechanism of Action
Dihydroxybergamottin exerts its effects primarily through the inhibition of the cytochrome P450 enzyme CYP3A4 . This enzyme is responsible for the oxidative metabolism of many drugs. By inhibiting CYP3A4, this compound increases the concentration of drugs that are substrates of this enzyme, thereby enhancing their bioavailability . The inhibition is mechanism-based, meaning that this compound binds to the enzyme and inactivates it through a covalent modification .
Comparison with Similar Compounds
Structural and Functional Classification
Furanocoumarins are categorized into linear (e.g., DHB, bergamottin) and angular (e.g., angelicin) subtypes. DHB belongs to Group IV furanocoumarins, characterized by epoxy or dihydroxy modifications of O-geranylated precursors. This structural distinction differentiates it from Group I compounds (e.g., bergamottin, aurapten), which lack these modifications .
Key Analogues and Their Bioactivities
Table 1: Comparative Analysis of DHB and Related Compounds
Mechanistic Differences
- DHB vs. Bergamottin : DHB is a substrate-independent, mechanism-based CYP3A4 inactivator, whereas bergamottin exhibits substrate-dependent reversible inhibition . DHB’s dihydroxy group enhances its binding affinity to CYP3A4’s heme iron, accelerating enzyme degradation .
- DHB vs. FC726: FC726, a furanocoumarin dimer, has a lower IC₅₀ (0.13 µM) but is scarce in natural sources. DHB’s broader distribution in grapefruit makes it clinically more relevant .
- DHB vs. Naringin: Naringin, a flavonoid, weakly inhibits CYP3A4 (IC₅₀ >100 µM) and contributes minimally to grapefruit-drug interactions compared to DHB .
Pharmacokinetic and Pharmacodynamic Variability
- DHB vs. Paradisin A : Paradisin A, a cyclic trimer, shows higher in vitro potency but lower stability and bioavailability than DHB .
- DHB vs. Peucedanin : Both exhibit antimicrobial activity, but DHB uniquely inhibits CYP3A4 due to its aliphatic side chain, which is absent in peucedanin .
Synergistic and Antagonistic Interactions
DHB synergizes with other furanocoumarins (e.g., bergamottin) to amplify CYP3A4 inhibition. However, Seville orange juice, despite containing bergaptol (a weak CYP3A4 inhibitor), lacks DHB and thus shows negligible drug interactions .
Biological Activity
Dihydroxybergamottin (DHB) is a furanocoumarin predominantly found in grapefruit juice and Seville orange juice, recognized for its significant biological activities, particularly its role as a potent inhibitor of cytochrome P450 3A4 (CYP3A4). This inhibition has important implications for drug metabolism and interactions, especially concerning medications metabolized by this enzyme.
Chemical Structure and Properties
This compound, specifically 6',7'-dihydroxybergamottin, exhibits a complex chemical structure that contributes to its biological functions. Its ability to interact with various enzymes makes it a subject of extensive pharmacological research.
CYP3A4 Inhibition
DHB is primarily known for its ability to inhibit CYP3A4, an enzyme responsible for the metabolism of approximately 50% of clinically used drugs. Studies have shown that DHB can significantly reduce the metabolic activity of CYP3A4 in human liver microsomes, leading to increased bioavailability of drugs that are substrates of this enzyme. The inhibition is characterized as time-dependent and mechanism-based, indicating that DHB modifies the enzyme's activity through a complex interaction rather than simple competitive inhibition.
- IC50 Values : Research indicates that the IC50 value for DHB against CYP3A4 ranges from 1 to 2 µM, demonstrating its potency compared to other known inhibitors like ketoconazole (IC50 ≈ 1.8 µM) and cimetidine (IC50 > 100 µM) .
Antioxidant Properties
While DHB is primarily studied for its enzyme inhibition capabilities, it also exhibits antioxidant properties. However, its effectiveness in scavenging free radicals appears limited compared to other compounds within the same class of furanocoumarins .
Herb-Drug Interactions
DHB is implicated in significant herb-drug interactions due to its presence in grapefruit juice. The compound can alter the pharmacokinetics of various drugs, leading to increased plasma concentrations and potential toxicity. This interaction is particularly crucial for drugs with narrow therapeutic indices such as statins and immunosuppressants .
Case Studies and Research Findings
Several studies have explored the implications of DHB in clinical settings:
- Impact on Drug Metabolism : A study involving healthy volunteers demonstrated that consumption of grapefruit juice containing DHB led to a marked increase in the bioavailability of midazolam, a drug metabolized by CYP3A4. This highlights the clinical relevance of DHB in dietary considerations for patients on certain medications .
- In Vitro Studies : Research utilizing HepG2 cells overexpressing CYP3A4 has provided insights into the molecular mechanisms by which DHB inhibits CYP3A4 activity. These studies confirmed that DHB not only inhibits CYP3A4 but also affects NADPH-cytochrome P450 reductase (POR) activity, further complicating its pharmacological profile .
Data Table: Summary of Biological Activities
Q & A
Basic Research Questions
Q. What experimental approaches are used to determine the inhibitory potency of DHB on cytochrome P450 enzymes?
Methodological Answer:
- Enzyme kinetics assays (e.g., time- and concentration-dependent inactivation studies) are critical. Use purified enzymes (e.g., CYP3A4, CYP1A1) in reconstituted systems with NADPH and cofactors to measure inactivation parameters (, ). For competitive inhibition, determine values via Lineweaver-Burk plots .
- Microsomal studies : Human or rat liver microsomes can assess isoform-specific inhibition using probe substrates (e.g., erythromycin for CYP3A4, ethoxyresorufin for CYP1A1). Include controls for mechanism-based inactivation (e.g., NADPH dependency) .
Q. How can researchers quantify DHB in biological samples during pharmacokinetic studies?
Methodological Answer:
- UPLC-MS/MS : Employ reverse-phase C18 columns with mobile phases (0.1% formic acid in water/acetonitrile) for separation. Validate methods using spiked plasma/urine samples, with detection limits <0.5 nmol/L .
- Sample preparation : Liquid-liquid extraction (ethyl acetate) or protein precipitation. Monitor DHB metabolites (e.g., bergaptol) in urine and plasma, as DHB’s distribution varies across matrices .
Q. What are the primary cytochrome P450 isoforms targeted by DHB, and how do inhibition constants (KiK_iKi) vary across species?
Methodological Answer:
- Human isoforms : CYP3A4 ( = 7.7 µM via mechanism-based inactivation) and CYP1A1 ( = 55 µM for competitive inhibition) are primary targets .
- Species differences : Rat CYP1A1 shows higher sensitivity ( = 1.72 µM) than human isoforms. Use cross-species microsomal comparisons to validate translational relevance .
Advanced Research Questions
Q. How do mechanism-based inactivation kinetics of DHB differ from competitive inhibition in CYP3A4 interactions?
Methodological Answer:
- Mechanism-based inactivation : Requires metabolic activation of DHB, leading to irreversible enzyme modification. Characterize using pre-incubation with NADPH, time-dependent activity loss, and heme retention assays (e.g., UV-Vis spectroscopy) .
- Competitive inhibition : Reversible binding without enzyme modification. Differentiate via determination under steady-state vs. pre-incubation conditions .
Q. What strategies resolve contradictory data on DHB’s selectivity between CYP1A1 and CYP3A4 isoforms?
Methodological Answer:
- Isoform-specific assays : Use recombinant CYP isoforms (e.g., baculovirus-expressed) to isolate activity. For example, DHB’s CYP1A1 inhibition in rats may not extrapolate to humans due to structural differences in enzyme active sites .
- Structural analogs : Compare DHB with bergamottin or epoxybergamottin to identify substituents (e.g., 6′,7′-dihydroxy groups) driving isoform specificity .
Q. What structural modifications of DHB analogs influence their inhibitory activity against P450 enzymes?
Methodological Answer:
- Geranyloxy chain modifications : Epoxidation (e.g., epoxybergamottin) reduces CYP3A4 affinity, while glycosylation (e.g., 6′-O-β-D-glucosyl derivatives) alters solubility and membrane permeability .
- Core structure : Furanocoumarin derivatives with 5-geranyloxy groups show stronger inhibition than C-prenylated analogs. Use molecular docking to predict binding interactions .
Q. What considerations are critical when extrapolating in vitro DHB-CYP interaction data to in vivo models?
Methodological Answer:
- Enterocyte vs. hepatic CYP3A4 : DHB’s bioavailability in grapefruit juice primarily inhibits intestinal CYP3A4, altering first-pass metabolism. Use portal vein-cannulated models to separate hepatic/intestinal contributions .
- Dose scaling : Account for species-specific clearance rates and protein binding. For example, rat studies may overpredict human effects due to higher metabolic capacity .
Q. How does DHB’s dual role as a substrate and inhibitor affect experimental design in drug interaction studies?
Methodological Answer:
- Auto-inhibition : Pre-incubate DHB with CYP enzymes to saturate metabolic pathways before adding co-administered drugs (e.g., felodipine). Monitor DHB depletion via LC-MS/MS .
- Time-course assays : Distinguish acute inhibition (reversible) from mechanism-based inactivation (irreversible) by varying incubation times .
Properties
IUPAC Name |
4-[(E,6R)-6,7-dihydroxy-3,7-dimethyloct-2-enoxy]furo[3,2-g]chromen-7-one | |
---|---|---|
Source | PubChem | |
URL | https://pubchem.ncbi.nlm.nih.gov | |
Description | Data deposited in or computed by PubChem | |
InChI |
InChI=1S/C21H24O6/c1-13(4-6-18(22)21(2,3)24)8-10-26-20-14-5-7-19(23)27-17(14)12-16-15(20)9-11-25-16/h5,7-9,11-12,18,22,24H,4,6,10H2,1-3H3/b13-8+/t18-/m1/s1 | |
Source | PubChem | |
URL | https://pubchem.ncbi.nlm.nih.gov | |
Description | Data deposited in or computed by PubChem | |
InChI Key |
IXZUPBUEKFXTSD-INMULRNOSA-N | |
Source | PubChem | |
URL | https://pubchem.ncbi.nlm.nih.gov | |
Description | Data deposited in or computed by PubChem | |
Canonical SMILES |
CC(=CCOC1=C2C=CC(=O)OC2=CC3=C1C=CO3)CCC(C(C)(C)O)O | |
Source | PubChem | |
URL | https://pubchem.ncbi.nlm.nih.gov | |
Description | Data deposited in or computed by PubChem | |
Isomeric SMILES |
C/C(=C\COC1=C2C=CC(=O)OC2=CC3=C1C=CO3)/CC[C@H](C(C)(C)O)O | |
Source | PubChem | |
URL | https://pubchem.ncbi.nlm.nih.gov | |
Description | Data deposited in or computed by PubChem | |
Molecular Formula |
C21H24O6 | |
Source | PubChem | |
URL | https://pubchem.ncbi.nlm.nih.gov | |
Description | Data deposited in or computed by PubChem | |
Molecular Weight |
372.4 g/mol | |
Source | PubChem | |
URL | https://pubchem.ncbi.nlm.nih.gov | |
Description | Data deposited in or computed by PubChem | |
CAS No. |
264234-05-1 | |
Record name | Dihydroxybergamottin | |
Source | ChemIDplus | |
URL | https://pubchem.ncbi.nlm.nih.gov/substance/?source=chemidplus&sourceid=0264234051 | |
Description | ChemIDplus is a free, web search system that provides access to the structure and nomenclature authority files used for the identification of chemical substances cited in National Library of Medicine (NLM) databases, including the TOXNET system. | |
Record name | DIHYDROXYBERGAMOTTIN | |
Source | FDA Global Substance Registration System (GSRS) | |
URL | https://gsrs.ncats.nih.gov/ginas/app/beta/substances/S2O194AWTV | |
Description | The FDA Global Substance Registration System (GSRS) enables the efficient and accurate exchange of information on what substances are in regulated products. Instead of relying on names, which vary across regulatory domains, countries, and regions, the GSRS knowledge base makes it possible for substances to be defined by standardized, scientific descriptions. | |
Explanation | Unless otherwise noted, the contents of the FDA website (www.fda.gov), both text and graphics, are not copyrighted. They are in the public domain and may be republished, reprinted and otherwise used freely by anyone without the need to obtain permission from FDA. Credit to the U.S. Food and Drug Administration as the source is appreciated but not required. | |
Retrosynthesis Analysis
AI-Powered Synthesis Planning: Our tool employs the Template_relevance Pistachio, Template_relevance Bkms_metabolic, Template_relevance Pistachio_ringbreaker, Template_relevance Reaxys, Template_relevance Reaxys_biocatalysis model, leveraging a vast database of chemical reactions to predict feasible synthetic routes.
One-Step Synthesis Focus: Specifically designed for one-step synthesis, it provides concise and direct routes for your target compounds, streamlining the synthesis process.
Accurate Predictions: Utilizing the extensive PISTACHIO, BKMS_METABOLIC, PISTACHIO_RINGBREAKER, REAXYS, REAXYS_BIOCATALYSIS database, our tool offers high-accuracy predictions, reflecting the latest in chemical research and data.
Strategy Settings
Precursor scoring | Relevance Heuristic |
---|---|
Min. plausibility | 0.01 |
Model | Template_relevance |
Template Set | Pistachio/Bkms_metabolic/Pistachio_ringbreaker/Reaxys/Reaxys_biocatalysis |
Top-N result to add to graph | 6 |
Feasible Synthetic Routes
Disclaimer and Information on In-Vitro Research Products
Please be aware that all articles and product information presented on BenchChem are intended solely for informational purposes. The products available for purchase on BenchChem are specifically designed for in-vitro studies, which are conducted outside of living organisms. In-vitro studies, derived from the Latin term "in glass," involve experiments performed in controlled laboratory settings using cells or tissues. It is important to note that these products are not categorized as medicines or drugs, and they have not received approval from the FDA for the prevention, treatment, or cure of any medical condition, ailment, or disease. We must emphasize that any form of bodily introduction of these products into humans or animals is strictly prohibited by law. It is essential to adhere to these guidelines to ensure compliance with legal and ethical standards in research and experimentation.