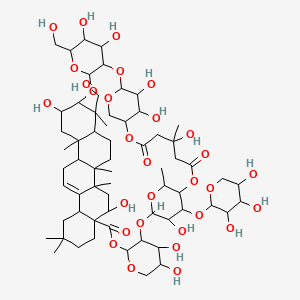
Tubeimoside II
Overview
Description
Mechanism of Action
- Its main role is to inhibit tumor growth, induce apoptosis (programmed cell death) in cancer cells, and suppress tumor invasion and metastasis .
- Tubeimoside II interacts with several cellular components:
- This compound impacts multiple pathways:
- Cellular Effects : It promotes cancer cell death, inhibits proliferation, and modulates immune responses .
Target of Action
Mode of Action
Biochemical Pathways
Pharmacokinetics
Result of Action
Action Environment
Biochemical Analysis
Biochemical Properties
Tubeimoside II plays a crucial role in various biochemical reactions. It interacts with several enzymes, proteins, and other biomolecules, influencing their activity and function. One of the primary interactions of this compound is with the enzyme caspase-3, which is involved in the execution phase of apoptosis. This compound activates caspase-3, leading to programmed cell death in cancer cells . Additionally, this compound interacts with the protein Bcl-2, a key regulator of apoptosis. By downregulating Bcl-2, this compound promotes apoptosis in cancer cells . The compound also affects the activity of the enzyme topoisomerase II, which is essential for DNA replication and repair. This compound inhibits topoisomerase II, leading to DNA damage and cell death .
Cellular Effects
This compound exerts significant effects on various types of cells and cellular processes. This compound also influences cell signaling pathways, such as the MKK4-p38α axis, which plays a critical role in cell survival and apoptosis . This compound has been shown to suppress tumor growth by inducing methuosis in a xenograft mouse model of hepatocellular carcinoma . Furthermore, this compound affects gene expression by modulating the activity of transcription factors and other regulatory proteins . It also impacts cellular metabolism by altering lipid metabolism, particularly cholesterol biosynthesis .
Molecular Mechanism
The molecular mechanism of this compound involves several key processes. At the molecular level, this compound binds to and activates caspase-3, leading to the cleavage of various substrates and the execution of apoptosis . The compound also inhibits the activity of topoisomerase II, resulting in DNA damage and cell death . This compound downregulates the expression of Bcl-2, promoting apoptosis in cancer cells . Additionally, this compound induces methuosis by hyperactivating the MKK4-p38α axis and boosting lipid metabolism, especially cholesterol biosynthesis . These molecular interactions highlight the multifaceted mechanism of action of this compound.
Temporal Effects in Laboratory Settings
In laboratory settings, the effects of this compound change over time. The compound exhibits stability under standard storage conditions, with minimal degradation observed over extended periods . In in vitro studies, the induction of apoptosis and methuosis by this compound occurs within hours of treatment, with significant cell death observed after 24 to 48 hours . Long-term effects of this compound include sustained suppression of tumor growth and reduced metastasis in animal models . These findings suggest that this compound maintains its efficacy over time, making it a valuable therapeutic agent.
Dosage Effects in Animal Models
The effects of this compound vary with different dosages in animal models. At low doses, this compound effectively induces apoptosis and methuosis in cancer cells without causing significant toxicity . At higher doses, this compound can cause adverse effects, such as hepatotoxicity and nephrotoxicity . The therapeutic window of this compound is relatively narrow, necessitating careful dose optimization to maximize its antitumor effects while minimizing toxicity . Studies have shown that combining this compound with other chemotherapeutic agents can enhance its efficacy and reduce the required dosage .
Metabolic Pathways
This compound is involved in several metabolic pathways, particularly those related to lipid metabolism. The compound has been shown to boost cholesterol biosynthesis by upregulating the expression of key enzymes involved in this pathway . Additionally, this compound affects the activity of enzymes involved in fatty acid metabolism, leading to alterations in metabolic flux and metabolite levels . These metabolic changes contribute to the overall antitumor effects of this compound by disrupting cellular homeostasis and promoting cell death .
Transport and Distribution
This compound is transported and distributed within cells and tissues through various mechanisms. The compound is soluble in dimethyl sulfoxide and methanol but insoluble in water . This compound interacts with specific transporters and binding proteins that facilitate its uptake and distribution within cells . Once inside the cell, this compound accumulates in specific compartments, such as the cytoplasm and lysosomes . This localization is crucial for its activity, as it allows this compound to interact with its target enzymes and proteins effectively .
Subcellular Localization
The subcellular localization of this compound plays a significant role in its activity and function. This compound is primarily localized in the cytoplasm and lysosomes, where it exerts its effects on cellular processes . The compound’s localization is mediated by specific targeting signals and post-translational modifications that direct it to these compartments . In the cytoplasm, this compound interacts with enzymes and proteins involved in apoptosis and DNA repair . In lysosomes, this compound induces methuosis by disrupting lysosomal membrane integrity and promoting vacuolization . These subcellular interactions are essential for the overall efficacy of this compound as an antitumor agent.
Preparation Methods
Synthetic Routes and Reaction Conditions
The synthesis of Tubeimoside-B involves several steps, starting from oleanolic acid, a common triterpenoid. The process includes glycosylation reactions to attach sugar moieties to the triterpenoid backbone. Typical reaction conditions involve the use of glycosyl donors and acceptors, with catalysts such as Lewis acids to facilitate the glycosylation .
Industrial Production Methods
Industrial production of Tubeimoside-B primarily relies on extraction from Bolbostemma paniculatum. The plant material is subjected to solvent extraction, followed by chromatographic purification to isolate Tubeimoside-B. This method ensures the compound’s high purity and is more cost-effective compared to synthetic routes .
Chemical Reactions Analysis
Types of Reactions
Tubeimoside-B undergoes various chemical reactions, including:
Oxidation: Tubeimoside-B can be oxidized to form different derivatives, which may exhibit altered biological activities.
Reduction: Reduction reactions can modify the functional groups on the triterpenoid backbone, potentially leading to new compounds with unique properties.
Substitution: Substitution reactions, particularly glycosylation, are crucial for modifying the sugar moieties attached to the triterpenoid core.
Common Reagents and Conditions
Oxidation: Common oxidizing agents include potassium permanganate and chromium trioxide.
Reduction: Reducing agents such as sodium borohydride and lithium aluminum hydride are used.
Substitution: Glycosylation reactions often use glycosyl donors like trichloroacetimidates and catalysts such as boron trifluoride etherate.
Major Products
The major products formed from these reactions include various glycosylated derivatives of Tubeimoside-B, each with potentially different biological activities .
Scientific Research Applications
Tubeimoside-B has a wide range of applications in scientific research:
Chemistry: It serves as a model compound for studying triterpenoid saponins and their chemical properties.
Biology: Tubeimoside-B is used to investigate cellular processes, including apoptosis and cell cycle regulation.
Medicine: Its antitumor and anti-inflammatory properties make it a candidate for developing new therapeutic agents. Research has shown its potential in treating cancers, inflammatory diseases, and viral infections.
Industry: Tubeimoside-B is explored for its use in developing natural pesticides and other agricultural applications .
Comparison with Similar Compounds
Tubeimoside-B is compared with other triterpenoid saponins such as:
Tubeimoside-A: Exhibits similar biological activities but with different potency and toxicity profiles.
Tubeimoside-C: Shows stronger anti-inflammatory and antitumor activities but higher acute toxicity compared to Tubeimoside-B.
Oleanolic Acid: The parent compound of Tubeimoside-B, widely studied for its hepatoprotective and anti-inflammatory properties .
Tubeimoside-B stands out due to its balanced profile of high biological activity and relatively low toxicity, making it a promising candidate for further research and development.
Biological Activity
Tubeimoside II (TBMS II), a triterpenoid saponin extracted from the tuber of Bolbostemma paniculatum, has garnered attention for its significant biological activities, particularly in the fields of oncology and immunology. This article delves into the biological activities of TBMS II, highlighting its mechanisms of action, effects on various cancer types, and comparative studies with other tubeimosides.
Overview of this compound
- Chemical Classification : Oleanane-type triterpenoid saponin
- Source : Extracted from Bolbostemma paniculatum
- Key Activities : Anti-inflammatory, antitumor, and antiviral properties
-
Induction of Apoptosis :
- TBMS II activates caspase-3, a critical enzyme in the apoptotic pathway, leading to programmed cell death in various cancer cells.
- It promotes cell cycle arrest in the G2/M phase, inhibiting cancer cell proliferation.
- Inhibition of Tumor Growth :
- Suppression of Metastasis :
Comparative Efficacy with Other Tubeimosides
A comparative analysis among tubeimosides I, II, and III has revealed that:
- Efficacy : TBMS II exhibits stronger anti-inflammatory and antitumor activities than TBMS I but is less toxic. Conversely, TBMS III shows even higher potency but with increased toxicity .
- Structure-Activity Relationship : The presence of a hydroxyl group at C-16 in TBMS II enhances its biological activity while reducing toxicity compared to its analogs .
Study 1: Antitumor Effects on Hepatocellular Carcinoma
- Methodology : Mice were treated with TBMS II (4 mg/kg/day) and tumor sizes were measured over time.
- Results : Significant reduction in tumor size was observed alongside histological evidence of methuosis in treated groups .
Study 2: Inhibition of Retinoblastoma Metastasis
- Methodology : Human retinoblastoma cell lines were treated with TBMS II.
- Results : The treatment led to decreased cell adhesion and migration through inhibition of TGF-β1 signaling pathways .
Data Table: Biological Activity Comparison
Compound | Anti-inflammatory Activity | Antitumor Activity | Toxicity Level |
---|---|---|---|
Tubeimoside I | Moderate | Moderate | Higher |
This compound | Strong | Strong | Lower |
Tubeimoside III | Very Strong | Very Strong | Highest |
Properties
IUPAC Name |
7,8,18,28,29,35,51,55,56,58-decahydroxy-30,54-bis(hydroxymethyl)-13,18,37,41,48,48,53,54-octamethyl-57-(3,4,5-trihydroxyoxan-2-yl)oxy-3,5,10,12,15,21,24,26,31,33-decaoxadecacyclo[39.9.3.211,14.222,25.134,38.01,46.04,9.027,32.037,42.045,53]octapentacont-44-ene-2,16,20-trione | |
---|---|---|
Source | PubChem | |
URL | https://pubchem.ncbi.nlm.nih.gov | |
Description | Data deposited in or computed by PubChem | |
InChI |
InChI=1S/C63H98O30/c1-25-46-47(89-51-43(77)38(72)29(67)21-82-51)45(79)53(85-25)91-48-39(73)30(68)22-83-54(48)93-56(80)63-14-13-57(2,3)15-27(63)26-9-10-34-59(5)16-28(66)50(60(6,24-65)33(59)11-12-61(34,7)62(26,8)17-35(63)69)92-55-49(42(76)40(74)31(20-64)87-55)90-52-44(78)41(75)32(23-84-52)86-36(70)18-58(4,81)19-37(71)88-46/h9,25,27-35,38-55,64-69,72-79,81H,10-24H2,1-8H3 | |
Source | PubChem | |
URL | https://pubchem.ncbi.nlm.nih.gov | |
Description | Data deposited in or computed by PubChem | |
InChI Key |
GBWAAJJGXQJTTA-UHFFFAOYSA-N | |
Source | PubChem | |
URL | https://pubchem.ncbi.nlm.nih.gov | |
Description | Data deposited in or computed by PubChem | |
Canonical SMILES |
CC1C2C(C(C(O1)OC3C(C(COC3OC(=O)C45CCC(CC4C6=CCC7C(C6(CC5O)C)(CCC8C7(CC(C(C8(C)CO)OC9C(C(C(C(O9)CO)O)O)OC1C(C(C(CO1)OC(=O)CC(CC(=O)O2)(C)O)O)O)O)C)C)(C)C)O)O)O)OC1C(C(C(CO1)O)O)O | |
Source | PubChem | |
URL | https://pubchem.ncbi.nlm.nih.gov | |
Description | Data deposited in or computed by PubChem | |
Molecular Formula |
C63H98O30 | |
Source | PubChem | |
URL | https://pubchem.ncbi.nlm.nih.gov | |
Description | Data deposited in or computed by PubChem | |
Molecular Weight |
1335.4 g/mol | |
Source | PubChem | |
URL | https://pubchem.ncbi.nlm.nih.gov | |
Description | Data deposited in or computed by PubChem | |
Q1: What is the structure of Tubeimoside II and how does it relate to its biological activity?
A: this compound is a cyclic bisdesmoside belonging to the oleanane type of triterpenoid saponins. [, , , , ] While its full spectroscopic data requires further collation from the literature, its structure notably features a C-16 hydroxyl group, distinguishing it from Tubeimoside I. This hydroxyl group plays a crucial role in enhancing the biological activity of this compound and reducing its toxicity compared to Tubeimoside I. [, ]
Q2: How does this compound exert its anti-tumor effects?
A: Research suggests that this compound exhibits its anti-tumor activity through multiple mechanisms. It has been shown to induce cell cycle arrest and programmed cell death (apoptosis) in various cancer cell lines. [] Specifically, this compound can promote tumor cell stagnation in the G2/M phase of the cell cycle, thereby inhibiting proliferation. [] Additionally, studies indicate that this compound can inhibit TGF-β1-induced metastatic progression in human retinoblastoma cells by suppressing redoxosome-dependent EGFR activation. []
Q3: Are there any studies comparing the efficacy of Tubeimosides I, II, and III?
A: Yes, comparative studies have revealed a structure-activity relationship among these three Tubeimosides. This compound demonstrates stronger anti-inflammatory, anti-tumor, and anti-tumorigenic activities compared to Tubeimoside I, while also exhibiting lower toxicity. [] Similarly, Tubeimoside III shows even greater potency than this compound but comes with increased toxicity. [] These findings highlight the potential of this compound as a promising candidate for cancer chemoprevention and chemotherapy. []
Q4: What analytical techniques are used to identify and quantify this compound in plant material?
A: Researchers utilize High-Performance Liquid Chromatography coupled with Electrospray Tandem Mass Spectrometry (HPLC-ESI/MS) for the identification and quantification of this compound. [] Additionally, a novel and sensitive method employing High-Performance Liquid Chromatography - Evaporative Light Scattering Detection (HPLC-ELSD) has also been developed for analysis. [] These techniques allow for the accurate determination of this compound content in Rhizoma Bolbostematis samples, ensuring quality control of this traditional medicine.
Q5: Has the natural variation in Tubeimoside content been investigated?
A: Studies analyzing 15 batches of Rhizoma Bolbostematis from various habitats have confirmed that the content of Tubeimosides, including this compound, can vary significantly depending on the source. [] This variability highlights the necessity for stringent quality control measures during the preparation and clinical application of Rhizoma Bolbostematis.
Disclaimer and Information on In-Vitro Research Products
Please be aware that all articles and product information presented on BenchChem are intended solely for informational purposes. The products available for purchase on BenchChem are specifically designed for in-vitro studies, which are conducted outside of living organisms. In-vitro studies, derived from the Latin term "in glass," involve experiments performed in controlled laboratory settings using cells or tissues. It is important to note that these products are not categorized as medicines or drugs, and they have not received approval from the FDA for the prevention, treatment, or cure of any medical condition, ailment, or disease. We must emphasize that any form of bodily introduction of these products into humans or animals is strictly prohibited by law. It is essential to adhere to these guidelines to ensure compliance with legal and ethical standards in research and experimentation.