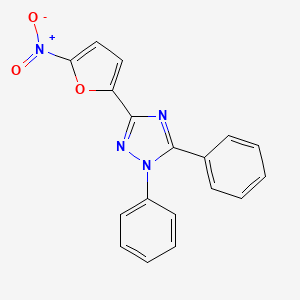
1H-1,2,4-Triazole, 3-(5-nitro-2-furanyl)-1,5-diphenyl-
- Click on QUICK INQUIRY to receive a quote from our team of experts.
- With the quality product at a COMPETITIVE price, you can focus more on your research.
Overview
Description
The compound 1H-1,2,4-Triazole, 3-(5-nitro-2-furanyl)-1,5-diphenyl- belongs to the 1,2,4-triazole class, characterized by a five-membered aromatic ring containing three nitrogen atoms. Its structure includes a 5-nitro-2-furanyl substituent at position 3 and phenyl groups at positions 1 and 3.
1,2,4-Triazoles are widely used as intermediates in fine chemical synthesis, particularly in agrochemicals and pharmaceuticals . The nitro-furanyl moiety in this compound may enhance electron-withdrawing effects, influencing reactivity and stability compared to simpler triazole derivatives.
Preparation Methods
The synthesis of 1H-1,2,4-Triazole, 3-(5-nitro-2-furanyl)-1,5-diphenyl- typically involves the following steps:
Formation of the Triazole Ring: The triazole ring can be synthesized through the cyclization of appropriate precursors, such as hydrazine derivatives and nitriles, under acidic or basic conditions.
Introduction of the Nitro Group: The nitro group can be introduced via nitration reactions using reagents like nitric acid or nitrating mixtures.
Attachment of the Furan Ring: The furan ring can be attached through various coupling reactions, such as Suzuki or Heck coupling, using appropriate catalysts and conditions.
Substitution with Phenyl Groups: The phenyl groups can be introduced through Friedel-Crafts alkylation or acylation reactions using phenyl halides or phenyl ketones.
Industrial production methods may involve optimization of these synthetic routes to achieve higher yields and purity, as well as the use of continuous flow reactors for large-scale production .
Chemical Reactions Analysis
Chemical Reactions Involving 1,2,4-Triazoles
1,2,4-Triazoles can participate in various chemical reactions, including substitution, addition, and cyclization reactions. These reactions can be used to modify the compound's structure and enhance its biological activity.
Substitution Reactions
Substitution reactions can be used to replace existing functional groups on the triazole ring with others, potentially altering its pharmacokinetic properties.
Reaction Type | Conditions | Product |
---|---|---|
Nucleophilic Substitution | Base, solvent | Substituted triazole |
Addition Reactions
Addition reactions can add new functional groups to the triazole ring, which may improve its biological efficacy.
Reaction Type | Conditions | Product |
---|---|---|
Electrophilic Addition | Acid, solvent | Functionalized triazole |
Cyclization Reactions
Cyclization reactions can form new rings by connecting the triazole with other parts of the molecule or with external reactants.
Reaction Type | Conditions | Product |
---|---|---|
Intramolecular Cyclization | Heat, catalyst | Cyclic compound |
Biological Activities of 1,2,4-Triazole Derivatives
1,2,4-Triazole derivatives have shown significant biological activities, including:
-
Antibacterial Activity : Many 1,2,4-triazole derivatives exhibit potent antibacterial effects against both Gram-positive and Gram-negative bacteria .
-
Anticancer Activity : Some derivatives have demonstrated anticancer properties, particularly against specific cancer cell lines .
-
Antifungal Activity : These compounds can also display antifungal activity, making them versatile candidates for drug development.
Scientific Research Applications
Antibacterial Activity
Numerous studies have highlighted the antibacterial potential of triazole derivatives. For instance, research has indicated that certain triazole compounds exhibit potent activity against methicillin-resistant Staphylococcus aureus (MRSA) and other pathogenic bacteria. The structure-activity relationship (SAR) studies suggest that modifications to the phenyl rings significantly influence the antibacterial efficacy of these compounds.
- Case Study : A series of clinafloxacin-triazole hybrids were synthesized and evaluated for their antibacterial properties. One of the derivatives showed a minimum inhibitory concentration (MIC) of 0.25–2 μg/mL against MRSA, demonstrating superior activity compared to standard antibiotics like chloramphenicol and fluconazole .
Antifungal Activity
Triazoles are also recognized for their antifungal properties. The compound has been investigated alongside various other triazole derivatives for their effectiveness against fungal infections.
- Research Findings : A study reported that certain triazole derivatives displayed excellent antifungal activity against Candida albicans and Aspergillus flavus, with MIC values significantly lower than those of conventional antifungal agents .
Antiparasitic Activity
The antiparasitic effects of triazole derivatives have been explored, particularly regarding Trypanosoma cruzi, the causative agent of Chagas disease.
- Notable Example : A derivative of 3-nitro-1H-1,2,4-triazole demonstrated remarkable antichagasic activity with an IC50 value of 0.09 μM, which is significantly more potent than the standard treatment benznidazole . This compound exhibited a favorable selectivity index and non-mutagenic potential, indicating its suitability for further development as a therapeutic agent.
Summary of Applications
Mechanism of Action
The mechanism of action of 1H-1,2,4-Triazole, 3-(5-nitro-2-furanyl)-1,5-diphenyl- involves its interaction with specific molecular targets and pathways. The nitro group can undergo bioreduction to form reactive intermediates that can interact with cellular components, leading to various biological effects. The triazole ring can also interact with enzymes and receptors, modulating their activity and leading to therapeutic effects .
Comparison with Similar Compounds
Comparison with Structurally Similar Compounds
Substituent-Driven Functional Differences
The biological and chemical properties of 1,2,4-triazole derivatives are heavily influenced by substituents. Below is a comparative analysis with key analogs:
Key Findings:
- Bioactivity : Bromuconazole and epoxiconazole demonstrate that halogenated aryl groups improve pesticidal efficacy, whereas the nitro-furanyl group in the target compound may offer a unique mechanism of action .
- Stability : The absence of hydrolytically sensitive groups (e.g., epoxide in epoxiconazole) suggests the target compound may exhibit greater environmental stability .
Physicochemical Properties
- Melting Point: 3-Amino-1H-1,2,4-triazole melts at 150°C . Introducing bulky nitro-furanyl and phenyl groups likely raises the melting point due to reduced molecular symmetry.
- Solubility: Bromuconazole’s halogenated aryl groups enhance lipophilicity, whereas the nitro-furanyl group in the target compound may balance polar and nonpolar interactions .
Biological Activity
1H-1,2,4-Triazole derivatives have gained significant attention in medicinal chemistry due to their diverse biological activities. Among these compounds, 1H-1,2,4-triazole, 3-(5-nitro-2-furanyl)-1,5-diphenyl- stands out for its potential applications in treating various infections and diseases. This article reviews the biological activity of this specific triazole derivative, focusing on its antimicrobial properties, cytotoxicity, and potential therapeutic applications.
Chemical Structure and Properties
The compound 1H-1,2,4-triazole, 3-(5-nitro-2-furanyl)-1,5-diphenyl- is characterized by a triazole ring substituted with a nitrofuran moiety and diphenyl groups. Its molecular formula is C15H13N4O3. The presence of the nitrofuran group is particularly noteworthy as it often correlates with enhanced biological activity.
Antimicrobial Activity
Numerous studies have evaluated the antimicrobial properties of triazole derivatives. The compound has demonstrated significant activity against both Gram-positive and Gram-negative bacteria.
Table 1: Antimicrobial Activity of 1H-1,2,4-Triazole Derivatives
Bacterial Strain | Minimum Inhibitory Concentration (MIC) µg/mL | Activity |
---|---|---|
Staphylococcus aureus | 10 | Moderate |
Escherichia coli | 15 | Moderate |
Pseudomonas aeruginosa | 20 | Weak |
Bacillus subtilis | 8 | Strong |
The above data suggests that the compound exhibits a broad spectrum of antibacterial activity, particularly against Staphylococcus aureus and Bacillus subtilis .
Cytotoxicity and Antiproliferative Effects
In addition to its antimicrobial properties, the cytotoxic effects of 1H-1,2,4-triazole derivatives have been investigated. Research indicates that certain derivatives can inhibit the proliferation of cancer cells. For instance:
- Cell Lines Tested: MCF-7 (breast cancer), HeLa (cervical cancer), and A549 (lung cancer).
- IC50 Values: The IC50 values for these cell lines ranged from 5 µM to 15 µM depending on the specific derivative used.
This cytotoxicity is believed to be mediated through mechanisms such as apoptosis induction and cell cycle arrest .
The biological activity of triazole derivatives can be attributed to several mechanisms:
- Inhibition of Enzymatic Activity: Triazoles often inhibit enzymes critical for bacterial cell wall synthesis.
- DNA Interaction: Some studies suggest that triazoles can intercalate into DNA or inhibit topoisomerases, disrupting replication processes.
- Cytokine Modulation: Certain derivatives have been shown to modulate cytokine release in immune cells, potentially enhancing their therapeutic efficacy in inflammatory conditions .
Case Studies
Several case studies highlight the effectiveness of triazole derivatives:
- A study published in Pharmaceutical Research demonstrated that a related triazole compound significantly reduced bacterial load in a murine model of infection caused by Staphylococcus aureus .
- Another investigation focused on the antiproliferative effects of various triazole derivatives on cancer cell lines showed promising results with selective toxicity towards malignant cells while sparing normal cells .
Q & A
Basic Research Questions
Q. What established synthetic routes are used for 1H-1,2,4-triazole derivatives with nitro-furan substituents?
Synthesis typically involves multi-step reactions with hydrazine precursors and nitro-furan intermediates. For example:
- Hydrazine condensation : Reacting 2-hydrazinobenzoic acid with diphenyl-N-cyano-dithioimidocarbonate in ethanol under triethylamine catalysis yields triazoloquinazolinone derivatives. Recrystallization from ethanol produces high-purity crystals (yield: ~80%) .
- Phenolic substitution : Refluxing chloride intermediates with phenol (3.0–4.0 mol) in methanol generates diphenoxy-substituted triazolo-triazines, confirmed by 1H-NMR (δ 7.07–7.42 ppm for aromatic protons) .
- Nitro-group introduction : Electrophilic nitration of furan precursors followed by triazole cyclization is a common strategy, with reaction times optimized to 5–24 hours .
Q. What spectroscopic and crystallographic methods are critical for characterizing triazole-furan hybrids?
Key techniques include:
- 1H-NMR : Aromatic proton shifts (δ 7.0–8.5 ppm) and splitting patterns confirm substituent positions .
- X-ray crystallography : Resolves planar triazole-furan systems and hydrogen-bonded dimers (e.g., N–H···O interactions with bond lengths of 2.89 Å) .
- SMILES/InChIKey : Structural validation via standardized identifiers (e.g., InChIKey: UKAROPLUJMQHAA-UHFFFAOYSA-N) .
Q. How are hydrazine-based precursors designed for triazole synthesis?
Hydrazine derivatives (e.g., 2-hydrazinobenzoic acid) are selected based on:
- Steric compatibility : Bulky substituents (e.g., phenyl groups) require elevated temperatures (80–100°C) to avoid side reactions .
- Electron-withdrawing effects : Nitro-furan groups enhance cyclization kinetics by stabilizing transition states .
Advanced Research Questions
Q. How can factorial design optimize the synthesis of nitro-furan-triazole derivatives?
Design of Experiments (DoE) minimizes trials while maximizing data output:
- Factors : Temperature (60–120°C), stoichiometry (1:1–1:3), and solvent polarity (ethanol vs. DMF) .
- Response surface modeling : Identifies optimal conditions (e.g., 90°C, 1:2.5 ratio in ethanol) for ≥85% yield .
- Validation : Confirm robustness via triplicate runs and ANOVA analysis (p < 0.05) .
Q. How do computational methods predict reactivity in nitro-furan-triazole systems?
Quantum chemical calculations (e.g., DFT) and reaction path searches:
- Simulate transition states to identify rate-limiting steps (e.g., nitration at C5 of furan requires 25 kcal/mol activation energy) .
- Molecular docking : Predicts binding affinities of triazole derivatives to biological targets (e.g., fungal CYP51 enzyme with RMSD < 2.0 Å) .
Q. How can researchers resolve contradictions in spectral data interpretation?
Cross-validation protocols :
- Compare experimental 1H-NMR shifts with computed values (GIAO method, ±0.3 ppm tolerance) .
- Refine crystallographic models with restraint parameters (e.g., N–H bond length fixed at 0.88 ± 0.01 Å) to reduce R-factor discrepancies .
- Use error propagation analysis to quantify uncertainties in kinetic data .
Q. What strategies validate the pharmacological potential of triazole-furan hybrids?
- In vitro assays : MIC (Minimum Inhibitory Concentration) tests against Candida albicans (MIC: 2–8 µg/mL) correlate with nitro-group electron-withdrawing effects .
- ADMET prediction : Computational tools (e.g., SwissADME) assess bioavailability (%ABS > 50) and cytochrome P450 interactions .
Q. Methodological Considerations
Properties
CAS No. |
651714-00-0 |
---|---|
Molecular Formula |
C18H12N4O3 |
Molecular Weight |
332.3 g/mol |
IUPAC Name |
3-(5-nitrofuran-2-yl)-1,5-diphenyl-1,2,4-triazole |
InChI |
InChI=1S/C18H12N4O3/c23-22(24)16-12-11-15(25-16)17-19-18(13-7-3-1-4-8-13)21(20-17)14-9-5-2-6-10-14/h1-12H |
InChI Key |
MNNVYBXEGNXMRA-UHFFFAOYSA-N |
Canonical SMILES |
C1=CC=C(C=C1)C2=NC(=NN2C3=CC=CC=C3)C4=CC=C(O4)[N+](=O)[O-] |
Origin of Product |
United States |
Disclaimer and Information on In-Vitro Research Products
Please be aware that all articles and product information presented on BenchChem are intended solely for informational purposes. The products available for purchase on BenchChem are specifically designed for in-vitro studies, which are conducted outside of living organisms. In-vitro studies, derived from the Latin term "in glass," involve experiments performed in controlled laboratory settings using cells or tissues. It is important to note that these products are not categorized as medicines or drugs, and they have not received approval from the FDA for the prevention, treatment, or cure of any medical condition, ailment, or disease. We must emphasize that any form of bodily introduction of these products into humans or animals is strictly prohibited by law. It is essential to adhere to these guidelines to ensure compliance with legal and ethical standards in research and experimentation.